What Is Work In Physics? Definition, Units, And Significance
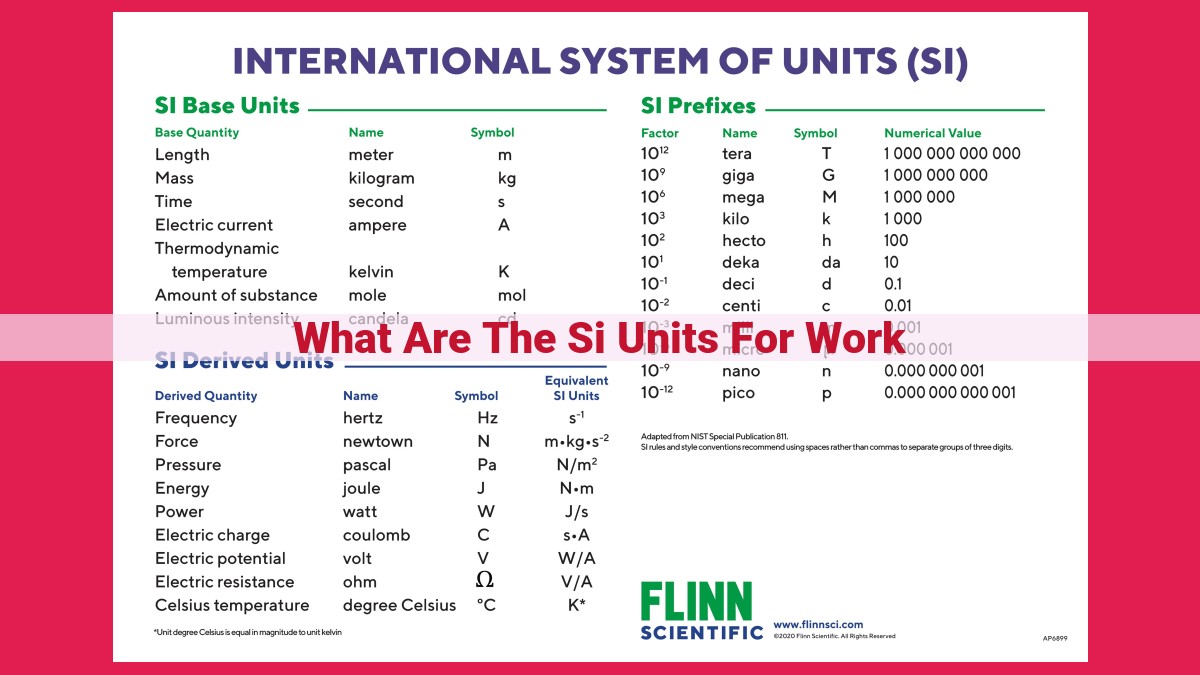
The SI unit of work is the joule (J), which is defined as the work done when a force of one newton is applied over a distance of one meter. Work is a measure of the energy transferred from one object to another due to the application of force over a distance. It is closely related to other concepts in physics, such as force, distance, energy, power, and kinematics. The mathematical definition of work is Work = Force × Distance × Cosine(angle), with units of newtons, meters, and radians. Other commonly used units of work include the foot-pound, horsepower-hour, and kilowatt-hour. Examples of work include lifting an object, pushing a box, and generating electricity. Work is a fundamental concept in physics, as it helps us understand energy transfer, power, and the motion of objects.
Definition of Work
- Explain that work is a fundamental concept in physics and define it as the energy transferred from one object to another due to the application of force over a distance.
What is Work in Physics?
In the realm of physics, where unraveling the mysteries of the universe is an ongoing pursuit, the concept of work plays a pivotal role. Work, in its essence, represents the energy transfer from one object to another, meticulously orchestrated by the application of force over a specific distance.
Imagine a scenario where you exert a force to lift a heavy object from the ground. As you maneuver the object upwards, you’re essentially performing work. This work, measured in units of joules, quantifies the energy utilized to counteract the gravitational pull and elevate the object against the force of resistance.
The joule, the SI unit of work, bears its name in honor of the esteemed physicist James Prescott Joule. One joule is defined as the work done when a force of one newton is applied over a distance of one meter.
Work is deeply intertwined with other fundamental concepts in physics, such as force, distance, energy, power, and kinematics. Force represents the agent that initiates change in motion, while distance measures the spatial displacement of an object. Energy, the capacity to do work, finds its expression in various forms, including kinetic energy and potential energy. Power, measured in watts, quantifies how rapidly work is done, and kinematics unveils the nuances of motion, delving into concepts like velocity and acceleration.
The Joule: Quantifying Work in Physics
In the realm of physics, work is a fundamental concept that describes the transfer of energy from one object to another due to the application of a force over a distance. The SI unit for work, named after the renowned physicist James Prescott Joule, is the joule (J).
A joule is defined as the work done when a force of one newton is applied to an object, causing it to move a distance of one meter in the direction of the applied force. In essence, the joule represents the amount of energy transferred during this specific interaction.
To illustrate, imagine lifting a 10-kilogram box from the ground to a height of 1 meter. The force required to lift the box is its weight, which is 10 newtons. Since the box moves a distance of 1 meter, the work done is calculated as follows:
Work = Force × Distance
Work = 10 N × 1 m
Work = 10 J
Hence, the work done in lifting the box is 10 joules. This energy has been transferred from your muscles to the box, allowing it to gain potential energy.
Understanding the joule as the unit of work is crucial in physics. It enables us to quantify the energy transfer involved in various processes, such as the contraction of muscles, the rotation of a wheel, or the flow of electricity. By measuring work in joules, we gain insights into the energy balance of systems and the efficiency of energy transformations.
Related Concepts and the Interplay of Work
Work, as defined in physics, is the energy transferred from one object to another due to the application of force over a distance. This concept intertwines with various related concepts, each playing a pivotal role in the understanding of work’s nature and significance.
Force: The initial driving force that causes an object to move. When a force acts upon an object, it can either cause it to accelerate, decelerate, or change its direction of motion.
Distance: The displacement or the length of the path traversed by an object under the influence of force. Distance is typically measured in meters or feet.
Energy: The ability to perform work. Energy exists in various forms, including kinetic energy (energy of motion), potential energy (stored energy), and thermal energy (energy due to heat). Work is a means of transferring energy from one object to another.
Power: The rate at which work is done or energy is transferred. Power is measured in watts (joules per second) and indicates the speed at which work is accomplished.
Kinematics: The study of the motion of objects without considering the forces that cause the motion. Kinematics provides insights into how objects move, including their velocity, acceleration, and displacement.
These concepts are intricately interconnected, forming a web of interactions that governs the behavior of objects in our physical world. Understanding these relationships is essential for comprehending the fundamental principles of physics and the practical applications of work in everyday life and technological advancements.
The Mathematical Essence of Work: Unraveling the Formula
In our exploration of the intricate world of physics, work emerges as a fundamental concept that describes the energy exchanged between objects. To fully grasp this enigmatic yet essential notion, let’s delve into the heart of its mathematical manifestation.
The cornerstone of the mathematical definition of work lies in the simple yet profound formula:
Work = Force × Distance × Cosine(angle)
Let’s dissect this formula, one variable at a time:
Force: Measured in newtons (N), force represents the push or pull exerted on an object. Imagine applying your muscular might to lift a heavy box; the force you exert is what propels the box upward.
Distance: This quantity, measured in meters (m), denotes the extent of an object’s displacement. In the case of the lifted box, distance refers to the vertical distance it travels from its initial to final position.
Cosine(angle): This trigonometric function, expressed as an angle in radians, captures the orientation of the force relative to the direction of displacement. When the force is applied parallel to the displacement, the cosine of the angle is 1, resulting in the maximum possible work.
By combining these variables, we arrive at the mathematical formula for work. It reveals that work is directly proportional to the magnitude of the force and the distance over which it acts, while also being influenced by the angle between the force and displacement vectors.
In its simplest form, work can be understood as the energy transferred from one object to another. When you push a heavy object, you exert a force over a distance, thereby transferring energy to the object. This energy manifests as the object’s increased velocity or displacement.
Example:
Consider the following scenario: you exert a force of 100 N to lift a box weighing 20 kg (approximately 44 lbs) vertically upward by a distance of 2 meters. The angle between the force and the displacement is 0 degrees (they are parallel). Using the formula, we can calculate the work done:
Work = Force × Distance × Cosine(angle)
Work = 100 N × 2 m × Cos(0°)
Work = 200 Joules
This calculation reveals that you have transferred 200 Joules of energy to the box, causing it to move upward.
Understanding the mathematical underpinnings of work is crucial for navigating the complexities of physics. It provides a precise and quantitative framework for analyzing energy transfer and motion. By mastering this formula and its implications, you will unlock a deeper comprehension of the physical world around you.
Relationship to Other Units
While the joule is the SI unit for work, various other units are commonly encountered. Let’s explore some of them and how they relate to the joule:
- Foot-pound (ft-lb): This unit is still used in some countries, particularly in engineering and construction. One foot-pound is the work done when a force of one pound is applied over a distance of one foot.
Conversion factor: 1 ft-lb = 1.356 J
- Horsepower-hour (hp-hr): This unit is often used to measure the energy output of engines and machines. One horsepower-hour is the work done when a force of one horsepower is applied over a distance of one hour.
Conversion factor: 1 hp-hr = 2.685 x 10^6 J
- Kilowatt-hour (kWh): This unit is commonly used in electrical applications to measure energy consumption. One kilowatt-hour is the work done when a power of one kilowatt is applied over a duration of one hour.
Conversion factor: 1 kWh = 3.6 x 10^6 J
Understanding these conversions is essential for practical applications. For instance, if a machine requires 500 ft-lb of work to operate, we can calculate its energy consumption in joules:
500 ft-lb x 1.356 J/ft-lb = 678 J
Similarly, if a motor has a power output of 10 hp, we can estimate the energy it can deliver in one hour:
10 hp x 2.685 x 10^6 J/hp-hr = 26.85 x 10^6 J
By being familiar with these units and conversions, we can seamlessly navigate various applications involving work and energy calculations.
Examples of Work in Our Daily Lives
Lifting an Object:
When you lift a book off a table, you are applying an upward force over a distance. The work done in this case is positive work because the force is in the same direction as the displacement. The amount of work done is equal to the force applied multiplied by the distance moved and the cosine of the angle between the force and the displacement.
Pushing a Box:
Imagine pushing a heavy box across the floor. The force you are applying is in the same direction as the box’s movement, making it positive work. The amount of work done depends on the magnitude of the force, the distance pushed, and the angle at which you push.
Generating Electricity:
Power plants use a variety of methods to generate electricity. One common method is through the use of turbines. When water or steam flows through a turbine, it applies a force on the turbine’s blades. This force causes the blades to rotate, which in turn drives a generator. The rotation of the generator creates an electric current, which is a type of positive work.
Other Examples:
Besides these common examples, work is present in many other activities:
- A carpenter driving a nail into wood
- A student writing an essay
- A swimmer overcoming water resistance
- A car accelerating on the road
In each of these scenarios, a force is applied over a distance, resulting in the transfer of energy. This transfer of energy is what defines work in physics.
The Significance of Work in Physics
In the realm of physics, work holds immense significance, playing a crucial role in our understanding of energy transfer, power, and the motion of objects. It forms the foundation of many fundamental concepts and applications in the physical world.
Understanding Energy Transfer
Work, defined as the transfer of energy from one object to another due to the application of force over a distance, is a key aspect of energy transfer. In various physical processes, work is done to change the energy state of objects. For instance, when you lift an object against gravity, you perform work by transferring energy to the object, increasing its potential energy.
Power and Efficiency
The concept of work is closely tied to power, which measures the rate at which work is done. Power, expressed in units of watts (joules per second), indicates how quickly energy is being transferred or converted. By understanding the work done and the time taken, we can assess the efficiency of systems and processes.
Motion and Kinematics
Work also plays a significant role in the study of motion and kinematics. According to Newton’s second law of motion, the work done on an object is equal to the change in its kinetic energy. This relationship forms the basis for analyzing the motion of objects under the influence of forces and understanding the mechanics behind everyday phenomena.
Applications in Engineering and Technology
The concept of work has found widespread applications in engineering and technology. From calculating the energy requirements of machines to designing efficient power systems, understanding work is essential for engineers and scientists. It helps them optimize designs, improve performance, and troubleshoot problems related to energy transfer.
In essence, work is a fundamental concept in physics that provides a framework for understanding the transfer of energy and the motion of objects. Its significance lies in its ability to quantify energy changes, determine power, analyze motion, and optimize systems across various domains of physics, engineering, and technology. By comprehending the concept of work, we gain a deeper appreciation of the intricate workings of the physical world and our ability to harness and manipulate energy effectively.