Work Hardening: Strengthening Metals For Enhanced Durability And Performance
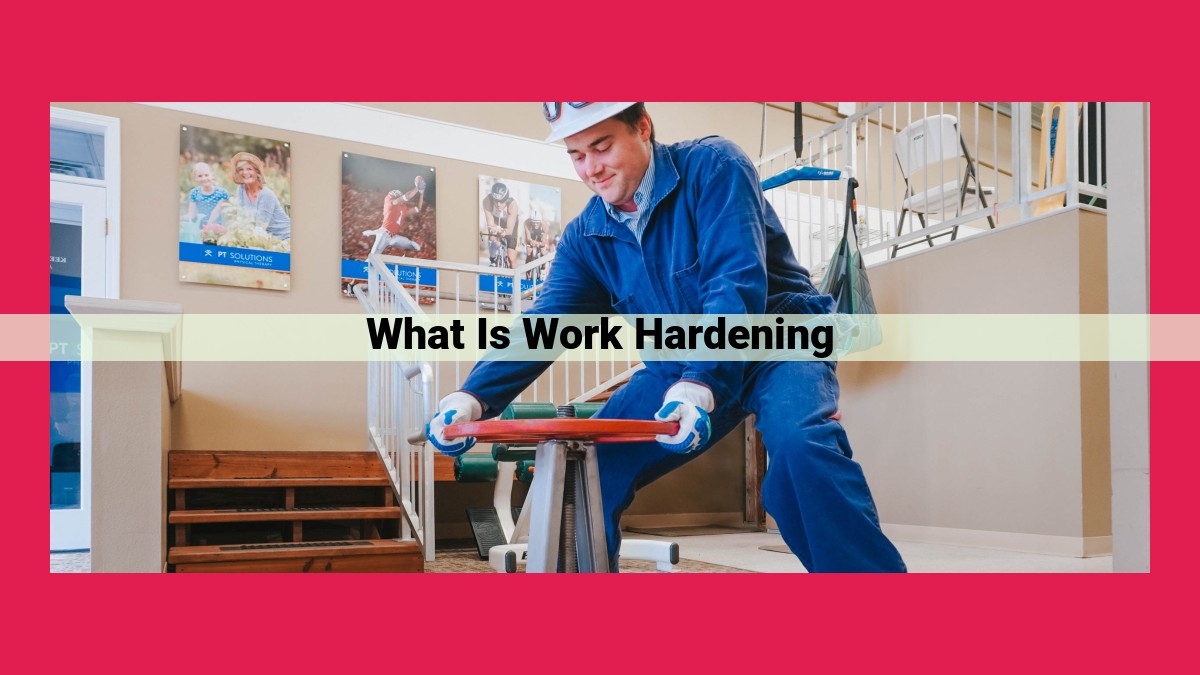
Work hardening is a process where metals increase their strength and hardness through plastic deformation. It involves the accumulation of dislocations during deformation, leading to an increase in resistance to further deformation. This process plays a critical role in material selection and engineering, as it allows for the tailoring of material properties to meet specific performance requirements. Applications of work hardening include enhancing the durability of metal components in various industries, such as automotive, aerospace, and manufacturing.
Work Hardening: Unlocking the Secrets of Material Strength
In the realm of material science, work hardening stands out as a transformative phenomenon that empowers materials with exceptional strength and durability. Imagine a metal object molded and shaped under intense pressure or hammering, emerging more robust than its initial state. This extraordinary transformation, known as work hardening, holds profound significance in the world of materials selection and engineering.
The Essence of Work Hardening
Work hardening, in its essence, is a process that occurs when a material undergoes plastic deformation. As the material is subjected to external forces, its internal structure undergoes a remarkable metamorphosis. Tiny imperfections within the material, called dislocations, multiply and interact, creating a network that impedes further deformation. This intricate dance of dislocations leads to an increase in strength and hardness.
The Impact on Material Properties
The effects of work hardening extend far beyond mere strength. It improves yield strength, which represents the stress at which a material begins to deform plastically, and tensile strength, which measures the maximum stress a material can withstand before it ruptures. Additionally, work hardening can influence a material’s ductility, its ability to deform before breaking.
Applications in the Real World
The practical applications of work hardening are vast and far-reaching. In metalworking operations, work hardening is harnessed to enhance material properties, enabling the creation of components with superior strength and durability. From automotive parts to construction materials, work hardening plays a pivotal role in ensuring their longevity and reliability.
Material Selection: A Tailored Approach
In the realm of material selection, work hardening takes center stage. By understanding the work hardening behavior of different materials, engineers can tailor material properties to meet specific design requirements. This enables the creation of components that not only withstand extreme loads but also maintain their integrity over time.
In conclusion, work hardening serves as a cornerstone of material science and engineering. Its ability to enhance material strength and durability has revolutionized the way we design and manufacture a wide range of products. By harnessing the power of work hardening, we can create components and structures that are not only robust but also enduring, paving the way for a world where materials perform flawlessly under the most demanding conditions.
Foundation Concepts of Work Hardening
Understanding the fundamental concepts underpinning work hardening is crucial for grasping its significance in materials science and engineering. These foundational principles revolve around plastic deformation, yield strength, tensile strength, and ductility—all intertwined with one another to shape the material’s behavior during work hardening.
Plastic Deformation: The Roots of Work Hardening
At the heart of work hardening lies the phenomenon of plastic deformation, a transformative process that occurs when a material undergoes permanent deformation under the influence of an applied force. Unlike elastic deformation, which allows a material to return to its original shape upon release of the force, plastic deformation leaves lasting changes in the material’s structure.
Yield Strength: The Threshold of Plasticity
Yield strength marks the pivotal point at which a material transitions from elastic behavior to plastic behavior. When a force exceeding the yield strength is applied, the material yields and undergoes plastic deformation. This transition marks a crucial milestone in the work hardening process.
Tensile Strength & Ductility: Indicators of Material Strength
Tensile strength measures the material’s resistance to pulling or stretching forces. It represents the maximum stress a material can withstand before it undergoes dramatic changes in shape or fractures. Ductility, on the other hand, gauges a material’s ability to deform plastically without breaking. A ductile material exhibits high ductility and can sustain significant elongation before fracturing.
The Interplay of Concepts: A Dance of Material Properties
The concepts of plastic deformation, yield strength, tensile strength, and ductility are intimately intertwined, shaping a material’s response to applied forces. Plastic deformation occurs beyond the yield strength, affecting the material’s tensile strength and ductility. This interplay forms the foundation of work hardening, ultimately governing the material’s strength and hardness.
Unveiling the Secrets of Work Hardening: How Materials Gain Strength
In the realm of materials science, the phenomenon of work hardening holds immense significance. It’s a process that enhances the strength and hardness of materials, making them more resilient and reliable for a wide range of applications.
The Mechanism Behind Work Hardening
At its core, work hardening is driven by the accumulation of dislocations within a material. Dislocations are imperfections in the crystal structure of a material that disrupt its regular atomic arrangement. When a material undergoes plastic deformation, these dislocations multiply and interact with each other.
As the number of dislocations increases, they encounter more obstacles, making it more difficult for the material to deform further. This increased resistance to deformation translates into an increase in the material’s strength and hardness.
Visualizing the Process
Imagine a field of soldiers marching in perfect formation. If an obstacle appears in their path, they must adjust their movements to navigate around it. Similarly, when a dislocation encounters another dislocation or an atomic defect, it must change its course.
As more dislocations accumulate, these obstacles become increasingly frequent, forcing the dislocations to interact and rearrange. This intricate dance of dislocations gives rise to the phenomenon of work hardening, ultimately making the material stronger and more resistant to deformation.
The Benefits of Work Hardening
The practical benefits of work hardening are far-reaching. By manipulating the number and distribution of dislocations within a material, engineers can tailor its mechanical properties to specific requirements.
This process enhances the material’s performance and durability, making it suitable for demanding applications such as automotive parts, aerospace components, and medical devices.
Work hardening is a powerful tool that enables materials scientists and engineers to control and enhance the properties of materials. By manipulating the dislocations within a material, they can create stronger, more durable, and more versatile materials that meet the challenges of modern engineering and industrial applications.
Applications of Work Hardening: Enhancing Strength and Durability
Work hardening, a phenomenon that strengthens materials through plastic deformation, finds numerous applications in shaping metals and enhancing the performance of engineering components.
Enhancing Material Properties in Metal Shaping Operations
In metalworking, work hardening plays a crucial role in shaping and strengthening materials. Processes such as cold working, where metals are deformed at room temperature without significant heating, capitalize on work hardening to improve material properties. For instance, cold rolling of steel increases its strength and hardness, making it suitable for applications such as automotive body panels and structural components.
Benefits for Engineering Components with High Strength and Durability Requirements
Engineering components often face demanding conditions, requiring high strength and durability. Work hardening provides a solution by increasing the strength of materials without compromising their ductility. This is particularly important for components subjected to wear, impact, or fatigue loads. For example, work-hardened gears exhibit increased resistance to wear, extending their lifespan and reducing maintenance costs.
Specific Examples and Industries Utilizing Work Hardening
Work hardening finds application in diverse industries. In the automotive sector, cold-rolled steel is used for body panels due to its enhanced strength and improved corrosion resistance. The aerospace industry relies on work hardening to strengthen aluminum alloys for aircraft components, reducing weight while maintaining structural integrity. In the medical field, work-hardened stainless steel is utilized in surgical instruments and implants due to its superior strength and resistance to corrosion.
By embracing the principles of work hardening, engineers and manufacturers unlock the potential to enhance material properties, improve component performance, and drive innovation in various industries.
Understanding the Role of Work Hardening in Material Selection
In the realm of material science and engineering, work hardening plays a crucial role in tailoring material properties to meet specific requirements. This process involves enhancing the strength and durability of metals through plastic deformation.
When a material undergoes plastic deformation, it experiences an accumulation of dislocations. These dislocations are tiny imperfections in the crystal structure that act as obstacles to further deformation. As the material deforms, the dislocations interact with each other, increasing the resistance to deformation. This increased resistance results in a higher yield strength, tensile strength, and hardness.
This enhanced strength and durability make work-hardened materials ideal for engineering components that require high performance and reliability. By carefully controlling the work hardening process, engineers can tailor the material properties to meet the specific demands of each application.
For example, in automotive manufacturing, work hardening is used to improve the strength of sheet metal used in body panels and chassis components. This increased strength enhances the durability of the vehicle and improves its resistance to damage. Similarly, in aerospace engineering, work-hardened aluminum alloys are used in aircraft structures due to their exceptional strength-to-weight ratio.
Understanding the role of work hardening in material selection is essential for engineers and designers seeking to optimize the performance and durability of their products. By leveraging this process, they can tailor material properties to meet specific requirements, ensuring that their creations stand the test of time and perform flawlessly under even the most demanding conditions.