The Wilson Cycle: Unraveling Earth’s Crust Evolution Through Geological Processes
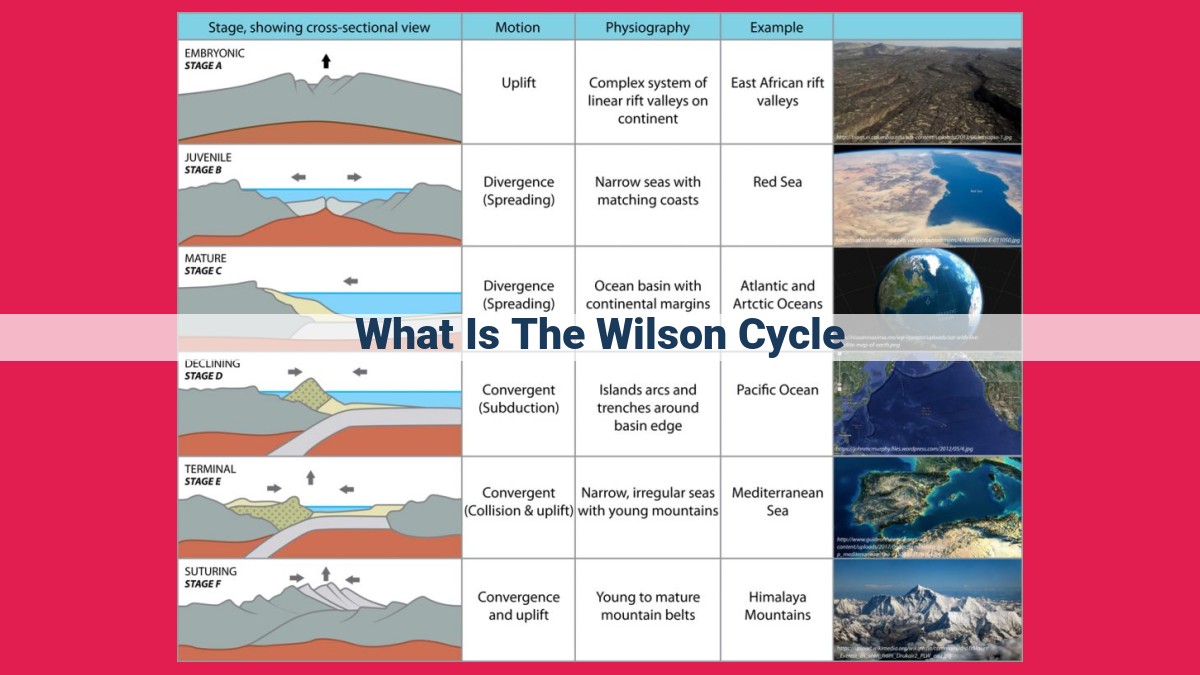
The Wilson Cycle is a conceptual framework describing the evolution of Earth’s crust through a continuous sequence of geological processes driven by plate tectonics. It involves subduction of oceanic crust, accretion of new crust to continents, orogeny for mountain building, and erosion and sedimentation for landscape sculpting. Metamorphism alters rocks during these processes. The Wilson Cycle is crucial for understanding Earth’s dynamic history and the formation of its geological features.
- Define the Wilson Cycle as a framework for understanding the evolution of Earth’s crust.
The Wilson Cycle: Unraveling the Evolution of Earth’s Crust
Our planet’s crust, the thin layer we inhabit, is a dynamic entity, constantly evolving and reshaping itself. The Wilson Cycle, a revolutionary framework proposed by geologist John Tuzo Wilson, provides a captivating tale of this ongoing transformation. It explains how Earth’s crust is created, recycled, and sculpted over millions of years.
Plate Tectonics: The Driving Force
At the heart of the Wilson Cycle lies plate tectonics, the dance of massive, interlocking slabs of Earth’s crust. These plates float on a molten layer of rock beneath the crust, drifting and colliding, shaping the landmasses we see today. The collision and separation of these plates drive the cycle of crustal evolution.
Subduction: The Catalyst
The story begins with subduction, where one tectonic plate slides beneath another. As the oceanic crust of the subducting plate plunges into the Earth’s mantle, it undergoes intense heat and pressure. This process melts the crust, creating molten rock that rises to form volcanoes and fueling the growth of accretionary wedges, piles of sediment and rock that accumulate on the overriding plate.
Plate Tectonics: The Foundation of the Wilson Cycle
In the vast tapestry of Earth’s geological history, the Wilson Cycle weaves an intricate masterpiece. This framework guides us through the dynamic evolution of our planet’s crust, a story driven by the ceaseless dance of plate tectonics.
Plate Tectonics: The Engine of Crustal Evolution
Imagine Earth’s lithosphere, the rocky outer layer of our planet, as a mosaic of massive, mobile plates. These tectonic plates float on the planet’s molten mantle, driven by the convection currents within. The relative motion of these plates, whether colliding, sliding past each other, or diverging, shapes the Earth’s surface and fuels the Wilson Cycle.
Continental Drift: A Transforming Landscape
One of the most profound concepts in plate tectonics is continental drift. In the early 20th century, Alfred Wegener proposed that the continents were once joined together in a supercontinent called Pangaea, which gradually sundered into the present-day configurations. The evidence for this continental drift is persuasive: matching fossil records, geologic formations, and ancient climates hint at a shared past.
Spreading Ridges: Birthing New Crust
At the boundaries where tectonic plates separate, spreading ridges emerge. Along these ridges, molten rock from the mantle erupts onto the seafloor, creating new oceanic crust. As the plates move away from the ridge, the newly formed crust cools and solidifies, widening the ocean basins.
Subduction Zones: The Crucible of Crustal Renewal
Where tectonic plates converge, one plate often descends beneath the other in a process called subduction. This sinking oceanic crust is dragged down into the Earth’s mantle, where it melts and releases volatiles into the overlying mantle. The molten material rises back to the surface, forming volcanic arcs and accretionary wedges on the overriding plate.
Subduction: The Catalyst for Crustal Renewal
In the grand tapestry of Earth’s geological evolution, subduction stands as a pivotal process, recycling oceanic crust and fueling the creation of new landmasses. Subduction is the process by which one tectonic plate descends beneath another, marking a critical juncture in the Wilson Cycle.
As oceanic crust travels across the globe, it converges with continental crust or other oceanic crust. At these boundaries, the denser oceanic plate is forced beneath the less dense continental plate, a process known as subduction. This descent triggers a cascade of geological transformations.
One profound consequence of subduction is the formation of volcanic arcs. As the oceanic crust sinks, it melts due to the intense heat and pressure in the mantle. This molten rock rises to the surface, forming volcanoes that create spectacular island chains or volcanic belts along the subduction zone.
Accretionary wedges are another byproduct of subduction. As the oceanic plate subducts, it scrapes against the continental plate, accumulating a wedge of sediments, volcanic rocks, and oceanic crustal fragments. Over time, these accreted materials become sutured to the continental margin, contributing to its growth and complexity.
Accretion: Building the Continents
- Discuss how subduction and accretion contribute to the growth and diversification of continental crust.
- Explore the formation of island arcs, ophiolites, and other accretionary complexes.
Accretion: Building the Continents
In the grand tapestry of plate tectonics, there exists a remarkable process known as accretion, the silent architect of Earth’s continents. Driven by the ceaseless dance of tectonic plates, it is a testament to the Earth’s ever-changing geology.
Imagine a subduction zone, where the heavy oceanic crust plunges beneath the lighter continental crust. As it descends, the oceanic crust undergoes a transformation, melted by the intense heat and pressure. This molten rock rises, forming volcanic arcs that become the seeds of new continental crust.
Over time, these volcanic arcs accumulate sediments that wash down from the eroding mountains. These sediments, together with the volcanic rock, are compressed and heated, metamorphosing into a diverse array of metamorphic rocks. As more material is added, the continent grows and matures.
Accretion also manifests in the formation of ophiolites, fragments of oceanic crust that have been thrust onto land. These ancient slices of the seafloor provide valuable insights into the evolution of Earth’s oceans.
This accretionary process is not confined to island arcs. It also occurs along the margins of continents, where fragments of oceanic crust are scraped off and accreted to the continental edge. These accretionary complexes, composed of mixtures of sedimentary, volcanic, and metamorphic rocks, tell the story of past collisions and tectonic interactions.
The Significance of Accretion
Accretion is not merely a geological curiosity; it is essential for the growth and diversification of continental crust. Without accretion, the continents would remain small and homogeneous, devoid of the rich mineral deposits, varied landscapes, and diverse ecosystems that characterize our planet today.
Through accretion, the Earth’s continents have grown and evolved over billions of years. They have witnessed the rise and fall of mountain ranges, the formation and break-up of supercontinents, and the gradual transformation of Earth’s surface. It is a testament to the dynamic and interconnected nature of our planet, where the forces of plate tectonics continuously shape and reshape the face of the Earth.
Orogeny: The Earth’s Sculptors
Throughout the eons, the Earth’s crust has been subjected to immense forces that have shaped its surface and created some of its most awe-inspiring features. One such force is orogeny, a process that has played a pivotal role in uplifting the mountains that dominate our landscapes.
The Forces Behind Orogeny
Orogeny is driven by the convergence of tectonic plates, massive slabs of the Earth’s crust that collide, slide against each other, or submerge beneath one another. As these plates interact, they exert colossal pressures on the rocks along their boundaries, triggering a series of geological transformations.
Folding, Faulting, and Metamorphism
When plates converge, the rocks caught between them are subjected to immense compression and shearing forces. This can cause the rocks to fold, forming intricate and often massive folds that can stretch for kilometers. Additionally, the faulting of rocks occurs when the stress exceeds their strength, creating fractures and displacements.
In addition to folding and faulting, orogeny also involves metamorphism. This process occurs when rocks are subjected to high heat and pressure, causing their mineralogy and texture to transform. Metamorphism can produce a wide range of metamorphic rocks, such as marble, schist, and gneiss.
The Birth of Mountain Belts
The combined effects of folding, faulting, and metamorphism can uplift the Earth’s crust, creating mountain belts. These belts are often characterized by rugged topography, steep slopes, and dramatic scenery. The Himalayas, the Andes, and the Alps are all examples of mountain ranges formed through the processes of orogeny.
The Significance of Orogeny
Orogeny has played a profound role in shaping the Earth’s surface. Mountain belts act as barriers to wind and precipitation, influencing climate patterns and creating diverse ecosystems. They also provide valuable resources, such as minerals, timber, and scenic beauty. Furthermore, the study of orogeny has helped scientists understand the dynamic nature of the Earth’s crust and the ongoing processes that continue to sculpt our planet’s surface.
Erosion and Sedimentation: Sculpting the Earth’s Tapestry
Erosion and sedimentation are the unsung heroes in the grand narrative of Earth’s geologic history. These processes relentlessly shape the planet’s surface, etching canyons, sculpting mountains, and creating the diverse landscapes we inhabit today.
Erosion, nature’s chisel, relentlessly carves away at rocks, soil, and vegetation. Wind, water, ice, and even gravity relentlessly assault the Earth’s surface, transforming it into a mosaic of landforms. Rivers carve deep valleys, glaciers gouge out landscapes, and the constant buffeting of waves chisels away at coastlines.
Sedimentation, the counterbalance to erosion, collects and deposits the eroded material. Rivers carry sediment to deltas and floodplains, winds pile it into sand dunes, and ocean currents distribute it along continental shelves. Over time, these sediments compact and solidify into sedimentary rocks, such as sandstone, limestone, and shale.
Erosion and sedimentation are not merely passive observers in Earth’s tectonic drama. They play an active role, influencing the very processes that reshape our planet. Sedimentation can add weight to the Earth’s crust, causing it to subsidence. This subsidence can trigger volcanism and alter plate tectonics by changing the distribution of mass on the Earth’s surface.
The interplay between erosion and sedimentation is a testament to the dynamic nature of the Earth. These processes are not only responsible for the ever-changing landscape but also shape the planet’s history and its future evolution. They are the sculptors of our planet, crafting the majestic peaks, rolling hills, and vast oceans that define our world.
Metamorphism: The Transformative Power of Heat and Pressure
Beneath Earth’s crust, where the relentless forces of geology hold sway, lies a realm of transformation. Here, in the depths of the Earth, rocks undergo a profound metamorphosis, sculpted by heat and pressure into new geological masterpieces.
Metamorphism, a process as ancient as the Earth itself, plays a vital role in shaping our planet’s crust. It occurs when existing rocks are subjected to extreme conditions, causing their mineralogy and texture to undergo dramatic changes.
Heat, the fiery breath of Earth’s core, drives this metamorphic dance. As temperatures soar, rocks begin to recrystallize, rearranging their molecular structure. Pressure then exerts its relentless grip, squeezing and deforming the recrystallized minerals into new, more compact forms.
The effects of metamorphism can be profound. Low-grade metamorphism creates rocks with a fine-grained texture, such as slate and phyllite. Intermediate-grade metamorphism produces lustrous schists and marbles, their minerals aligned in shimmering bands. High-grade metamorphism transforms rocks into gneisses with swirling, complex patterns, a testament to the intense heat and pressure they have endured.
The transformative power of metamorphism extends beyond the rocks themselves. By altering the composition and structure of the crust, metamorphism influences the movement of tectonic plates and the formation of mountain ranges. It is a process that continually reshapes our planet, leaving an enduring legacy in the rocks that tell the story of Earth’s geological past.