Why Carbon’s Bonding Powers Fuel Life As We Know It
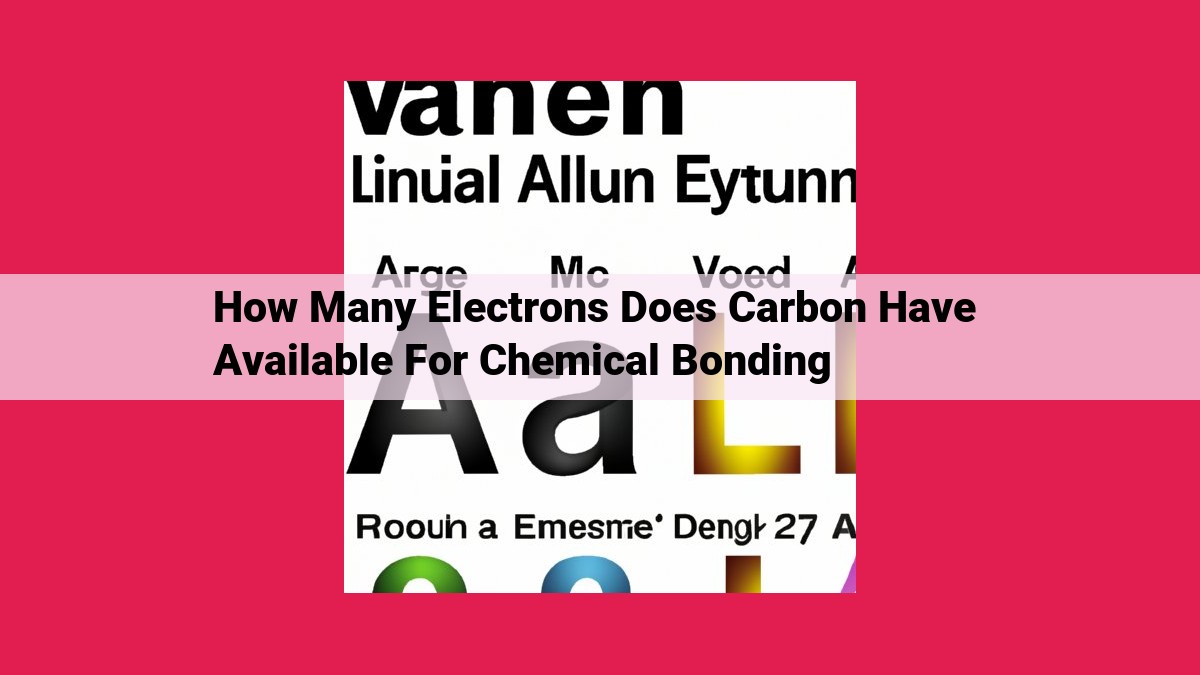
Carbon’s chemical bonding capabilities stem from its electronic configuration. Its four valence electrons occupy the outermost shell, making them available for chemical interactions. This key characteristic allows carbon to form covalent bonds with other atoms, sharing electron pairs to achieve a stable electronic configuration. The number and arrangement of these valence electrons influence carbon’s bonding behavior, enabling it to form diverse molecules with varying shapes and properties.
Understanding the Electronic Foundation of Carbon’s Chemical Bonding
As we delve into the fascinating world of chemistry, understanding the electronic configuration of elements plays a pivotal role in deciphering their chemical bonding capabilities. The electronic configuration of an atom refers to the arrangement of its electrons within its atomic orbitals. This arrangement dictates the chemical properties of the element, including its bonding capacity.
The Aufbau principle, named after Austrian physicist Julius Edgar Lilienfeld, states that electrons fill atomic orbitals in a specific sequence, starting with the lowest energy level and progressively moving to higher energy levels. Another crucial principle, Hund’s rule, proposes that electrons occupy orbitals of equal energy individually, maximizing their spins to achieve the lowest energy configuration.
By comprehending the electronic configuration of an element, we gain insights into its potential for chemical bonding. For instance, the number of valence electrons, those located in the outermost shell, directly influences the element’s bonding capacity. Carbon, the focus of our exploration, possesses four valence electrons, making it an incredibly versatile element capable of forming a vast array of compounds.
The Valence Shell and Valence Electrons: Understanding Carbon’s Reactivity
In the world of chemistry, the concept of valence electrons plays a pivotal role in unraveling the intricate tapestry of chemical bonding. These electrons reside in the outermost shell of an atom, eagerly waiting to engage in dance with other atoms, forming the glue that holds molecules together. For carbon, this is a particularly captivating tale, as its four valence electrons endow it with an exceptional capacity for bonding and shape-shifting in the realm of molecular architectures.
The valence shell, like a bustling city, is the outermost electron-occupied region of an atom, where the action unfolds. It’s a vibrant hub where chemical reactivity takes center stage. For carbon, this bustling city accommodates four energetic valence electrons, eager to venture beyond their atomic confines.
These four valence electrons are the key to carbon’s multifaceted bonding capabilities. They act like tiny magnets, attracting electrons from other atoms, forming covalent bonds that unite atoms into molecular entities. The number of valence electrons dictates the chemical bonding capacity of an element, and for carbon, with its quartet of valence electrons, the possibilities are boundless.
So, delve into this riveting account of carbon’s valence shell and valence electrons, as we unravel the secrets behind the intricate dance of chemical bonding and uncover the remarkable versatility of this elemental wonder.
Valence Electrons and the Magic of Chemical Bonding
In the realm of chemistry, the valence electrons of an atom play a crucial role in determining its chemical bonding capacity. These electrons, residing in the outermost energy level, are like the social butterflies of the atomic world, eager to interact and form bonds with other atoms.
The number of valence electrons an atom possesses directly influences its bonding capacity. For instance, carbon, with its four valence electrons, has the unique ability to form myriad types of compounds. This versatility stems from its capacity to share, gain, or lose electrons to achieve a stable electronic configuration.
Besides the number of valence electrons, the electronegativity difference between atoms also impacts the type and strength of the bonds formed. Electronegativity measures an atom’s affinity for electrons. When two atoms with significant electronegativity differences bond, the more electronegative atom pulls the electrons closer to itself, resulting in a polar covalent bond. In contrast, when atoms with similar electronegativities bond, the electrons are equally shared, forming a nonpolar covalent bond.
The interplay between valence electrons and electronegativity difference is like a delicate dance. It determines the types of bonds that form, their strength, and ultimately the properties of the resulting compounds. These principles lie at the heart of chemistry, shaping the molecular world we inhabit.
Hybridization and Molecular Orbitals: The Secret to Carbon’s Versatility
Hybridization: A Tale of Orbital Blending
Carbon’s ability to form diverse molecular structures stems from a remarkable phenomenon known as hybridization. This process involves the blending of atomic orbitals, creating new hybrid orbitals with unique properties. Think of it as a molecular makeover, where orbitals undergo a transformation to become more suitable dance partners for other atoms.
Formation of Hybrid Orbitals and Molecular Orbitals
When atomic orbitals hybridize, they combine their energies and shapes to form new, evenly spaced orbitals. These hybrid orbitals then interact with each other and with orbitals of neighboring atoms, forming covalent bonds. This interplay gives rise to molecular orbitals, the quantum mechanical description of how electrons behave within a molecule.
Hybridization and Molecular Geometry
The hybridization of carbon’s valence orbitals determines the geometry of carbon-based molecules. For instance, in methane (CHâ‚„), carbon’s valence electrons occupy tetrahedrally-shaped hybrid orbitals, resulting in the molecule’s tetrahedral structure. This precise alignment minimizes electron-electron repulsion, ensuring stability.
Diversifying Carbon Compounds
Hybridization empowers carbon with unparalleled versatility. By altering the hybridization of its valence orbitals, carbon can form a vast array of compounds with vastly different properties. This diversity underlies the immense range of organic molecules found in living organisms and countless synthetic materials.
Hybridization and molecular orbitals are fundamental concepts that underpin carbon’s chemical bonding capacity. Through this intricate interplay, carbon transcends its atomic configuration, giving rise to the myriad forms and functions of carbon-based compounds that shape our world.