Weight Measurement: Understand Newtons (N) In The Si System
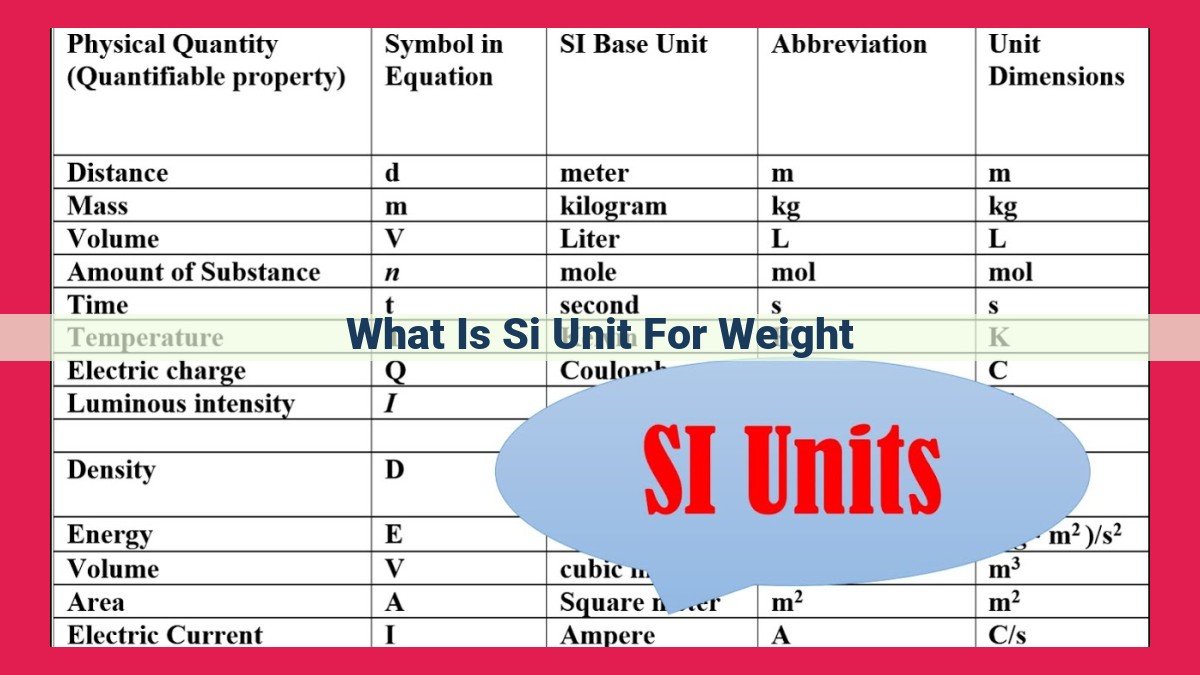
- Weight, the force exerted on an object due to gravity, is measured in Newtons (N) in the International System of Units (SI).
Understanding Weight: A Fundamental Force
Gravity’s Grip: Weight Unraveled
In the tapestry of the universe, gravity reigns supreme, exerting its invisible force upon every object within its reach. Weight, a fundamental manifestation of this force, plays a pivotal role in shaping our physical world.
Weight, defined as the force exerted on an object due to gravity, is an omnipresent phenomenon that affects objects of all sizes, from the tiniest specks of dust to the celestial giants that grace the night sky. It is the invisible tether that binds us to the Earth, preventing us from floating aimlessly into the void.
Gravity, the architect of weight, is a force that attracts objects towards each other. The greater the mass of an object, the more gravitational pull it exerts, and thus, the heavier it is. Conversely, objects with less mass experience a correspondingly weaker gravitational force, resulting in a lighter weight.
The concept of weight becomes even more intriguing when we consider that it is not an inherent property of an object. Rather, it is a varying force that depends on the strength of the gravitational field in which the object resides. For instance, an object on the surface of the Earth will weigh significantly more than the same object on the surface of the Moon, simply because the Earth’s gravitational pull is much stronger.
Mass vs. Weight: A Matter of Distinction
In the realm of physics, understanding the difference between mass and weight is crucial. While often used interchangeably, these two concepts hold distinct meanings.
Mass represents the amount of matter present in an object. It is an intrinsic property that remains constant regardless of its location or surroundings. Think of it as the essential substance that makes up the object, akin to the number of LEGO bricks in a toy car.
Weight, on the other hand, is a force exerted on an object due to the gravitational pull between the object and a celestial body, typically Earth. It depends not only on the mass of the object but also on the acceleration due to gravity at the object’s location.
Imagine a helium balloon filled with different amounts of air. The mass of each balloon is different, representing the amount of helium they contain. However, if you place these balloons on a scale on Earth, they will all have the same weight because they are experiencing the same gravitational pull from Earth (9.8 m/s²).
In essence, weight is the _product of mass and acceleration due to gravity_ (Weight = Mass × Acceleration due to Gravity). If you travel to the Moon, where the gravitational pull is weaker, the **weight of the same helium balloons will be significantly lower than on Earth, while their mass remains unchanged.
Introducing the Newton: The SI Unit of Weight
When it comes to measuring the force exerted on an object due to gravity, the world of physics relies on a fundamental unit: the Newton. Named after the renowned physicist Sir Isaac Newton, the Newton serves as the standard unit of force within the International System of Units (SI).
The Newton is defined as the force required to impart an acceleration of one meter per second squared (1 m/s²) to an object with a mass of one kilogram (1 kg). Essentially, it represents the amount of force that can cause a one-kilogram object to accelerate one meter per second every second.
The relationship between the Newton, mass, and acceleration can be expressed mathematically:
Force (Newtons) = Mass (kilograms) × Acceleration (meters per second squared)
or
N = kg × m/s²
This formula highlights how force is directly proportional to both mass and acceleration. An object with greater mass will experience a greater force of gravity, and an object subject to a higher acceleration due to gravity will also experience a greater force.
Understanding the Newton and its connection to mass and acceleration is crucial for comprehending the fundamental nature of weight and the forces that govern the physical world around us.
Weight and the Tug of Gravity
Weight, an invisible yet influential force, is a manifestation of the gravitational pull exerted on objects. Gravity, a captivating phenomenon, acts as a cosmic magnet, drawing objects towards each other. When focused on the Earth-bound objects, this gravitational pull manifests as acceleration due to gravity, a constant measured approximately as 9.8 meters per second squared (m/s²).
Acceleration due to gravity plays a pivotal role in determining the weight of an object. The greater the object’s mass, the stronger the gravitational pull and consequently, the heavier it becomes. Moreover, changes in gravitational force due to variations in altitude, latitude, and celestial bodies can cause weight to fluctuate. For instance, astronauts experience a weightlessness effect in space due to reduced gravitational forces.
The relationship between weight, mass, and acceleration due to gravity can be expressed through the formula:
Weight (N) = Mass (kg) × Acceleration due to Gravity (m/s²)
Understanding this relationship is crucial for comprehending the interplay between gravity, weight, and the behavior of objects. It’s a fundamental concept that finds practical applications in various fields, including physics, engineering, and our everyday lives.
Connecting the Dots: Weight, Mass, and Gravity
Imagine yourself standing on the surface of Earth, your feet firmly planted against the ground. An invisible force pulls you down, keeping you grounded and preventing you from floating into the vastness of space. This force, my friend, is what we call weight.
Weight is not to be confused with mass. Mass is the amount of matter that makes up your physical being, while weight is the force exerted on you due to the gravitational pull of the planet. As you venture to different celestial bodies, your weight may change, but your mass remains the same.
The relationship between weight, mass, and gravity is beautifully encapsulated in a simple mathematical formula:
Weight = Mass × Acceleration due to Gravity
Let’s break it down:
- Mass is measured in kilograms (kg). It represents the quantity of matter an object contains.
- Acceleration due to Gravity is denoted by g, and on Earth, it is approximately 9.8 meters per second squared (m/s²). This value represents the rate at which an object accelerates towards the center of the planet.
- Weight is the force that results from the interplay between mass and gravity. It is expressed in Newtons (N), the SI unit of force.
By understanding this formula, you gain the power to calculate the weight of any object, given its mass and the acceleration due to gravity at its location.
For instance, if you weigh 70 kg on Earth, your weight on the Moon would be significantly less, around 12 kg. This is because the Moon’s gravitational pull is weaker than Earth’s, resulting in a lower acceleration due to gravity.
The interplay between weight, mass, and gravity is a fundamental aspect of our universe. It influences everything from the way objects fall to the very structure of celestial bodies. Embracing this knowledge, we deepen our understanding of the physical world and our place within it.
The Importance of Gravity: Free Fall and Stellar Phenomena
Gravity, the invisible force that binds the universe together, plays a crucial role in countless phenomena, from the gentle sway of leaves to the cosmic ballet of distant galaxies. Its influence is most evident in the concept of free fall. When an object is in free fall, it is experiencing only the pull of gravity, without any other forces acting upon it.
One of the most iconic examples of free fall is the drop of an apple from a tree. As the apple falls, the only force acting on it is the downward pull of gravity, causing it to accelerate towards the Earth’s center. This acceleration is known as acceleration due to gravity, and it is a constant value of approximately 9.8 meters per second squared (m/s²) on the surface of our planet.
Free fall has been a subject of fascination for centuries, inspiring scientists like Galileo Galilei and Isaac Newton to unravel the secrets of gravity. In the 17th century, Galileo famously conducted experiments by dropping objects from the Leaning Tower of Pisa, demonstrating that all objects fall with the same acceleration regardless of their mass. This groundbreaking observation laid the foundation for Newton’s subsequent formulation of the law of universal gravitation.
The significance of gravity extends far beyond the simple act of falling objects. In the realm of astronomy, it governs the motion of planets around the Sun, galaxies within clusters, and even the expansion of the universe itself. Without the gravitational force holding celestial bodies together, the cosmos would be a chaotic and boundless expanse.
Gravity also plays a crucial role in various practical applications. Engineers rely on the principles of gravity to design bridges, buildings, and other structures that can withstand its relentless pull. In the field of medicine, gravity is utilized in diagnostic imaging techniques like magnetic resonance imaging (MRI) and X-rays, which exploit the differential effects of gravity on different tissues.
In essence, gravity is an omnipresent force that shapes our world in countless ways. From the fall of an apple to the celestial symphony of the stars, it is an integral part of our universe, providing order, stability, and a fascinating subject of scientific exploration.
Practical Applications: Weight in the Real World
Understanding weight is not just a theoretical concept; it has crucial applications in various fields and everyday life.
Engineering and Construction: Engineers rely on weight calculations to design structures, machines, and infrastructure. Determining the weight distribution is essential for ensuring stability and preventing collapse. In construction, weight calculations are used to calculate loads on buildings, bridges, and other structures, ensuring they can withstand gravitational forces.
Physics and Space Exploration: In physics, studying weight helps us understand the nature of gravity and motion. In space exploration, considering the weight of spacecraft, satellites, and astronauts is critical. Weight affects propulsion, trajectory calculations, and ensuring the safety and functionality of spacecraft in microgravity environments.
Everyday Life: Weight plays a significant role in our daily lives. Weighing ourselves helps us monitor our health and make informed decisions about our diet and exercise. In cooking and baking, we measure ingredients by weight to ensure the precise proportions required for recipes. Weight is also essential in shipping and logistics, as it determines shipping costs and packing requirements.
Examples of Weight’s Influence:
- On Earth:
- A heavy object, like a refrigerator, exerts a greater force on the floor and requires more effort to move.
- The weight of a car affects its fuel consumption and performance.
- The weight of a backpack affects the strain on a hiker’s shoulders.
- In Space:
- Astronauts experience weightlessness due to the reduced gravitational force in space.
- Satellites orbit the Earth because their weight and the Earth’s gravity create a balance.
- The weight of a spaceship affects its maneuverability and the amount of fuel required for propulsion.
Understanding weight allows us to harness its effects in practical applications, from designing stable structures to exploring the vastness of space. It is an essential concept that shapes our world and our daily experiences.