Understand Water’s Molecular Structure: Delving Into Lewis Structure And Molecular Geometry
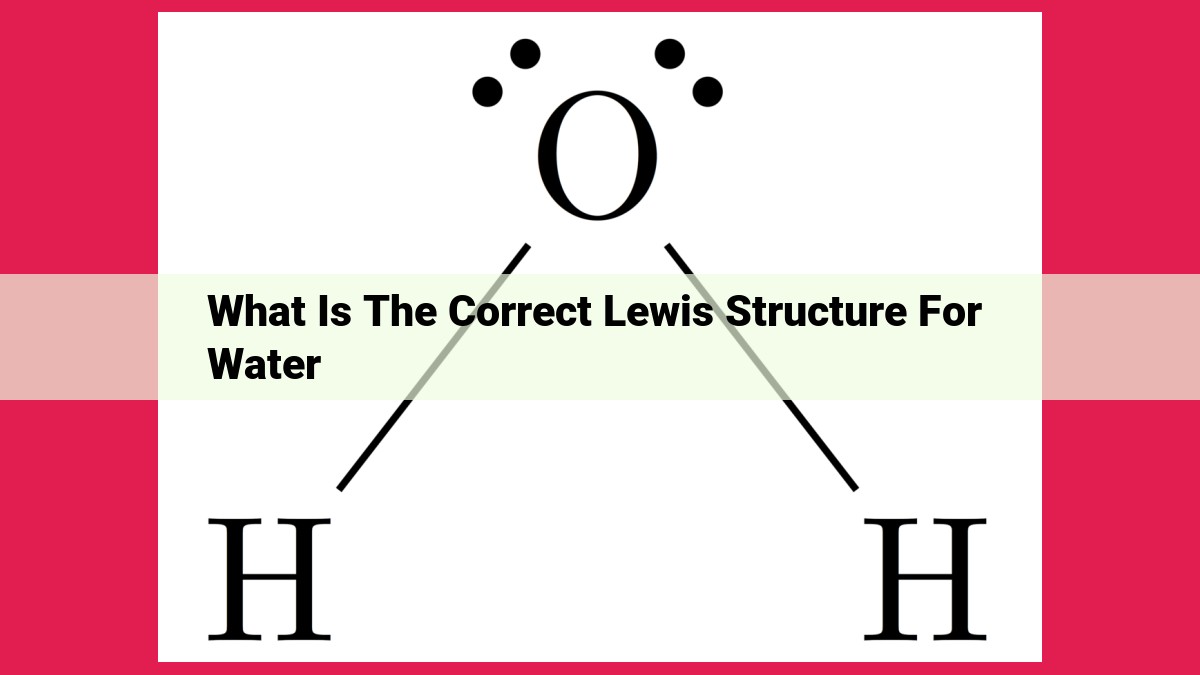
The correct Lewis structure of water depicts the arrangement of valence electrons to accurately represent its molecular geometry. Using VSEPR theory, the central oxygen atom forms two single bonds with hydrogen atoms. The electron-pair geometry is tetrahedral, but due to the presence of two lone pairs on the oxygen, the molecular shape becomes bent, forming an angle of approximately 104.5 degrees between the H-O-H bonds. This structure allows for a more stable molecular configuration by minimizing electron repulsion.
Imagine a tiny world where molecules dance and interact, each with a unique shape that governs its behavior. Molecular geometry is the fascinating realm that explores these shapes, revealing the profound impact they have on molecular properties.
Every molecule, no matter how small or complex, possesses a specific geometrical arrangement of atoms. This arrangement determines its bonding pattern, reactivity, intermolecular forces, and countless other characteristics that define its role in the grand scheme of life.
By understanding molecular geometry, we unravel the secrets of chemical reactions, predict the properties of new materials, and pave the way for advancements in various scientific disciplines. From drug design to nanotechnology, molecular geometry serves as a cornerstone for unlocking countless possibilities.
VSEPR Theory: Predicting Electron-Pair Geometry
- Describe the principles of VSEPR theory and how it predicts molecular shapes.
VSEPR Theory: Unveiling the Secret Behind Molecular Shapes
In the realm of molecular chemistry, molecular geometry reigns supreme, dictating the shape and properties of countless molecules that shape our world. VSEPR theory, an ingenious concept, serves as our guide to understanding and predicting these intricate molecular structures.
The Genius of VSEPR Theory
VSEPR theory, short for Valence Shell Electron Pair Repulsion theory, is a groundbreaking tool that allows us to decipher the spatial arrangement of electron pairs around an atom. It operates on the fundamental principle that electron pairs naturally repel each other, leading them to adopt the geometry that minimizes this repulsion.
Applying VSEPR to Predict Shapes
Armed with VSEPR theory, we can embark on a journey to predict the electron-pair geometry of a molecule. This geometry forms the foundation for determining the molecular shape. Here’s how it works:
- Step 1: Determine the Total Number of Valence Electrons
Count the valence electrons for all atoms in the molecule, including those involved in bonds and lone pairs.
- Step 2: Arrange Electron Pairs to Minimize Repulsion
Using Lewis structures, represent the electron pairs around the central atom. Arrange these pairs in a way that maximizes the distance between them.
- Step 3: Identify the Electron-Pair Geometry
Based on the arrangement of electron pairs, determine the electron-pair geometry. Common geometries include linear, trigonal planar, and tetrahedral.
Unlocking Molecular Shapes
Once the electron-pair geometry is established, we can confidently predict the molecular shape. The molecular shape describes the actual three-dimensional arrangement of atoms in the molecule. For instance, a molecule with trigonal planar electron-pair geometry adopts a planar triangular shape.
VSEPR: A Powerful Tool for Molecular Exploration
VSEPR theory is a testament to the ingenuity of scientific inquiry. It provides a systematic approach to understanding and predicting molecular geometry, paving the way for a deeper comprehension of chemical bonding and the behavior of molecules in various environments.
Lewis Structures: Unlocking the Secrets of Molecular Geometry
In the realm of chemistry, understanding the invisible world of molecules is crucial to unraveling their properties and behavior. One essential tool in this endeavor is the Lewis structure, a visual representation of how atoms bond and share electrons.
Defining Lewis Structures: A Rosetta Stone for Molecular Geometry
Lewis structures, named after their inventor, Gilbert Newton Lewis, provide a blueprint for the arrangement of electrons in a molecule. They depict atoms as circles and electrons as dots, with lines representing shared electron pairs. These diagrams are more than just visual aids; they hold the key to predicting a molecule’s geometry.
The Magic of VSEPR: Predicting Molecular Shapes
The Valence Shell Electron Pair Repulsion (VSEPR) theory uses Lewis structures to predict the shapes of molecules. VSEPR postulates that electron pairs around an atom repel each other, resulting in molecular geometries that minimize this repulsion. For example, a molecule with two electron pairs will adopt a linear shape, while one with three electron pairs will form a trigonal planar shape.
Connecting Lewis Structures and Molecular Geometry
The electron-pair geometry, as determined by the number of bonding and lone pair electrons, directly influences a molecule’s overall shape. For instance, a molecule with four bonding pairs and no lone pairs will have a tetrahedral geometry, while one with three bonding pairs and one lone pair will adopt a trigonal pyramidal shape.
Uncovering the Role of Lone Pairs: Invisible yet Influential
Lone pair electrons, which are not involved in bonding, can have a significant impact on molecular geometry. They occupy more space than bonding pairs and exert a stronger repulsive force, causing adjacent bonding pairs to be pushed farther apart. Consequently, the presence of lone pairs can alter the shape of a molecule, making it more distorted or asymmetrical.
Electron-Pair Geometry and Molecular Shape
How Electron Arrangement Dictates a Molecule’s Looks
As you dive into the captivating world of chemistry, you’ll discover that the arrangement of electrons around atoms plays a pivotal role in determining their molecular geometry. This geometry, in turn, significantly influences a molecule’s properties and behavior.
Visualizing Electron-Pair Geometry
Picture electrons as tiny magnets, each striving to occupy as much space as possible. This dance of electrons results in specific electron-pair geometries. For instance, two electron pairs arrange themselves in a linear fashion, forming 180-degree angles between them. Three electron pairs adopt a trigonal planar geometry, creating 120-degree angles, while four electron pairs form a tetrahedral shape with angles of 109.5 degrees.
Molecular Shape: Beyond Electron Pairs
The electron-pair geometry provides the framework for a molecule’s overall shape. But it’s not the whole story. Lone pair electrons, those not involved in bonding, can also influence molecular geometry. For example, a molecule with three electron pairs and one lone pair adopts a trigonal pyramidal shape due to the lone pair’s repulsive effect.
Double Bonds: Linearity Unraveled
Double bonds, where two pairs of electrons connect atoms, introduce an exciting twist. The presence of double bonds forces molecules to adopt linear shapes. This is because the electron pairs associated with double bonds repel each other more strongly, pushing atoms as far apart as possible, resulting in a straight-line configuration.
By understanding the interplay between electron-pair geometry and molecular shape, you’ll gain deeper insights into why molecules behave the way they do. This knowledge forms the foundation of your chemical exploration, empowering you to predict properties, understand reactions, and unlock the secrets of the chemical world.
The Role of Lone Pair Electrons in Molecular Geometry
Defining Lone Pairs
Lone pair electrons are pairs of valence electrons that are not involved in covalent bonding. They reside on specific atoms within molecules, influencing their spatial arrangement and molecular properties.
Lone Pairs and Molecular Shape
The presence of lone pairs affects the electron-pair geometry of a molecule, which in turn determines its molecular shape. Lone pairs repel bonding electron pairs, causing them to move as far away as possible. This repulsion results in bent or distorted molecular shapes compared to those without lone pairs.
Lone Pairs and Polarity
Lone pairs also contribute to the polarity of molecules. They create partially negative regions on the atoms they occupy, making the molecule polar. This polarity can influence intermolecular interactions, such as hydrogen bonding.
Importance of Lone Pairs
Understanding the role of lone pair electrons is crucial in predicting molecular geometry, shape, and properties. It allows chemists to predict and explain various phenomena, from the reactivity of molecules to their physical properties.
Lone pair electrons play a significant role in shaping the geometry and properties of molecules. Their presence influences bonding patterns, electron distribution, and molecular interactions. By considering the effects of lone pairs, we gain a deeper understanding of how molecules behave and interact with their environment.
Double Bonds and the Impact on Molecular Geometry
When atoms form chemical bonds, they share electrons to achieve stability. Single bonds are formed by sharing one pair of electrons, while double bonds involve the sharing of two electron pairs. This additional pair of electrons has a significant impact on molecular geometry.
Unlike single bonds that allow for a range of molecular shapes, double bonds enforce a more rigid linear geometry. The two electron pairs in a double bond repel each other strongly, forcing the atoms involved to adopt a straight line configuration. This linear shape minimizes the electron-electron repulsion.
Consider the molecule carbon dioxide (CO2). Each carbon atom forms a double bond with an oxygen atom. The result is a linear geometry, with the oxygen atoms positioned directly opposite each other and the carbon atom in the middle. This linear shape is essential for the molecule’s stability and its unique properties, such as its ability to absorb infrared radiation.
In summary, double bonds significantly affect molecular geometry by enforcing a linear arrangement. This linear geometry arises from the strong repulsion between the two electron pairs in the double bond, which forces the atoms involved to align in a straight line configuration. Understanding the impact of double bonds on geometry is crucial for predicting the shapes and properties of molecules, and this knowledge plays a vital role in various fields of science, including chemistry, biology, and materials science.
Unlocking the Secrets of Water’s Molecular Geometry
Imagine yourself as a detective, embarking on a captivating journey to unravel the intricate secrets of water’s molecular geometry. This exploration will illuminate its profound impact on the properties that make water the lifeblood of our planet.
As you delve into the realm of molecular geometry, you’ll discover VSEPR theory—the guiding force behind predicting electron-pair geometry, the blueprint for a molecule’s shape. Through the lens of Lewis structures, you’ll visualize the electronic connections within water, revealing how these connections dictate its geometry.
Unveiling the correct Lewis structure of water is akin to solving a tantalizing puzzle. The arrangement of lone pair electrons, those enigmatic electrons that don’t participate in bonding, plays a crucial role in shaping the molecule’s 3D structure.
Intriguingly, water’s two double bonds—those powerful connections between oxygen and hydrogen—induce a linear geometry. This unique arrangement grants water its exceptional polarity, enabling it to dissolve a vast array of substances.
Step by step, you’ll unravel the intricate relationship between electron-pair geometry and molecular shape, gaining invaluable insights into the behavior of water and countless other molecules that shape our world.