Unlocking The Versatility Of Transition Metals: Delving Into Valence Electrons And Chemical Reactivity
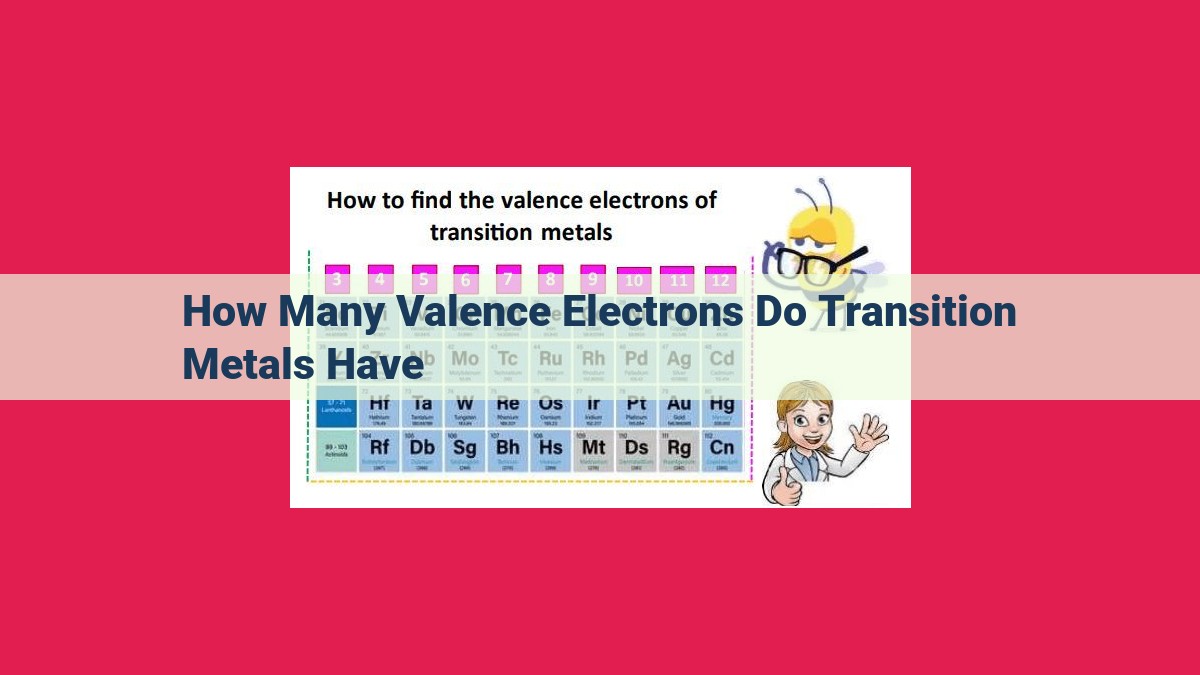
Transition metals, known for their diverse properties and applications, are characterized by variable valence electrons. Located in the d-block of the periodic table, these metals typically have d-orbitals that can hold up to 10 electrons. The number of valence electrons directly influences their chemical reactivity and bonding behavior, enabling them to form a wide range of compounds with varying oxidation states. Understanding the valence electron configuration of transition metals is essential for comprehending their unique properties and their impact in fields such as catalysis and materials science.
Transition Metals: Unlocking the Power of Electrons in the Heart of the Matter
In the realm of chemistry, a captivating group of elements known as transition metals holds a position of paramount importance, shaping countless aspects of our world. These metallic wonders, adorned with distinctive properties, have become indispensable in fields ranging from electronics to medicine. At the very heart of their remarkable abilities lies a dance of electrons, particularly those residing in the valence shell.
Valence Electrons: The Key to Understanding Transition Metals
The key to unlocking the secrets of transition metals lies in their valence electrons. These electrons, located in the outermost energy level, possess a unique ability to roam freely, enabling transition metals to form diverse chemical bonds. This versatility in bonding behavior gives rise to a mesmerizing array of properties that set them apart.
From Catalysis to Electronics: The Practical Magic of Transition Metals
The interplay of valence electrons in transition metals translates into a remarkable range of applications. Their ability to act as catalysts, facilitating countless chemical reactions, has revolutionized industries worldwide. In the world of electronics, transition metals serve as essential components in transistors and semiconductors, powering our digital devices and shaping the modern era.
In conclusion, transition metals stand as a testament to the profound impact of valence electrons on the properties and applications of elements. Their distinctive characteristics, rooted in the interplay between d-block elements, electron configurations, orbitals, and valence electrons, have paved the way for a myriad of technological advancements. As we delve deeper into the captivating world of transition metals, the interconnectedness of these concepts becomes increasingly evident, revealing the intricate tapestry of chemistry that shapes our world.
d-Block Elements: The Foundation of Transition Metals
The world of chemistry is filled with fascinating elements, each with its own unique set of properties. Among these, the transition metals stand out for their versatility and significance in various fields. But what exactly are transition metals, and how do they come into existence? The answer lies in the realm of d-block elements.
Unveiling d-Block Elements
In the periodic table, elements are arranged in vertical columns known as groups and horizontal rows called periods. The transition metals occupy the central section of the periodic table, spanning Groups 3 to 12. These elements are characterized by the presence of d-orbitals, which are a set of five orbitals with specific shapes and energy levels.
The Relationship to Transition Metals
The presence of d-orbitals is the defining feature that distinguishes d-block elements from other groups. Transition metals, by definition, are elements that possess partially filled d-orbitals. This unique electronic configuration gives rise to their characteristic properties, such as variable oxidation states, colorful ion formation, and the ability to form complexes with ligands.
Key Characteristics of d-Block Elements
D-block elements exhibit a fascinating array of properties that set them apart from other elements:
- Variable Oxidation States: Transition metals can exhibit multiple oxidation states, meaning they can lose or gain varying numbers of electrons. This versatility is attributed to the presence of multiple d-electrons that can be involved in chemical bonding.
- Formation of Colored Ions: When transition metal ions are dissolved in water or other solvents, they often absorb light of specific wavelengths, resulting in the formation of colored solutions. This phenomenon is due to electronic transitions within the d-orbitals.
- Complex Formation: Transition metals have a remarkable ability to form complexes with ligands, which are molecules or ions that donate electrons. These complexes play a vital role in biological processes, catalysis, and various technological applications.
The Role of Valence Electrons
The properties of d-block elements and transition metals are intimately linked to the number of valence electrons. Valence electrons are the electrons in the outermost energy level of an atom, which determine its chemical reactivity. In transition metals, the valence electrons reside in the d-orbitals and play a crucial role in bonding, oxidation states, and complex formation.
Understanding the relationship between d-block elements, transition metals, and valence electrons provides a deeper insight into the fascinating world of chemistry. These concepts lay the groundwork for exploring the applications and implications of transition metals in various fields, from catalysis to materials science and beyond.
Transition Metals: The Heart of the Matter
In the realm of chemistry, transition metals stand as a captivating group of elements that possess an unwavering charm. Their unique character, often described as the gateway to understanding the periodic table, stems from their exceptional electronic structure.
Dwelling within the d-block of the periodic table, transition metals are characterized by the presence of partially filled d-orbitals. This electronic configuration grants them a remarkable versatility, enabling them to bond with a wide array of atoms and molecules.
The number of d-electrons within a transition metal dictates its chemical behavior. Those with a higher number of d-electrons tend to form stronger bonds and exhibit higher reactivity, while those with a lower number of d-electrons exhibit weaker bonds and reduced reactivity. This variability in d-electron count underpins the extraordinary applications of transition metals in fields such as catalysis, materials science, and electronics.
Moreover, the d-orbitals of transition metals play a crucial role in their magnetic properties. The interaction between unpaired d-electrons gives rise to magnetism, a phenomenon that finds practical applications in magnetic materials, data storage, and MRI machines.
In essence, the versatile electronic structure of transition metals, particularly their partially filled d-orbitals and variable d-electron count, renders them indispensable to modern technology and our understanding of the chemical world.
Electron Configuration: Unraveling the Blueprint of Transition Metals
In the enchanting world of chemistry, transition metals stand out as captivating elements that hold the key to unlocking remarkable properties and applications. These enigmatic substances owe their distinctive character to the intricate arrangement of electrons within their atomic structure, a blueprint known as their electron configuration.
The Aufbau principle, a guiding light in the realm of chemistry, dictates the sequential filling of atomic orbitals as you venture from one element to the next. When it comes to transition metals, their story unfolds within the d-block, a special region in the periodic table where electrons reside in the d-orbitals. These orbitals, shaped like delicate lobes and dumbbells, play a pivotal role in determining the valence electrons—the electrons that eagerly participate in chemical reactions.
Electron configuration serves as a window into the inner workings of transition metals. By deciphering the precise arrangement of electrons within these orbitals, we can unravel the secrets of their chemical behavior and understand the diverse applications that make them indispensable in our modern world.
Orbitals: The Spatial Dimension of Electrons
Prepare to embark on a captivating journey into the realm of atomic orbitals, the enigmatic dance of electrons within the confines of atoms. These orbitals are the very foundation of transition metals, determining their character and shaping their chemical prowess. Picture a three-dimensional symphony, where electrons gracefully pirouette within their designated orbitals, each with its unique shape and energy.
Amongst the five d-orbitals, meet the dxy, dyz, dxz, dxy, and dz2. These ethereal shapes possess an intriguing variety, distinct from the familiar s and p orbitals. Their orientation in space forms a tetrahedral and an octahedral arrangement, providing an intricate lattice for the electrons to reside.
Now, delve into the intricate interplay between d-orbital energies and the number of valence electrons. Valence electrons, the outermost electrons, hold the key to understanding a transition metal’s reactivity. The energy levels of the d-orbitals dictate how many valence electrons an atom can accommodate, directly influencing its chemical behavior.
Through this journey of atomic orbitals, we unravel the profound connection between the spatial dimension of electrons and the essence of transition metals. These orbitals serve as the invisible stage upon which the captivating dance of electrons unfolds, shaping the myriad properties and applications that make transition metals indispensable in our technological world.
Valence Electrons: Unlocking the Secrets of Transition Metals
At the heart of chemistry lies a fascinating realm where transition metals reign supreme. These remarkable elements hold the key to diverse fields, from catalysis to electronics. Understanding their versatility begins with the exploration of their valence electrons.
Valence electrons are the outermost electrons in an atom’s electron configuration. They determine an element’s chemical behavior, and transition metals possess a unique characteristic: their d-block electrons can act as valence electrons. This variable valence allows them to exhibit multiple oxidation states, contributing to their unmatched versatility in chemical reactions.
The intricate relationship between d-block elements, electron configuration, orbitals, and valence electrons is a symphony of chemistry. D-block elements populate the rows of the periodic table where d-orbitals are filling. The number of valence electrons corresponds to the number of unpaired electrons in these d-orbitals. This interplay governs the chemical reactivity and properties of transition metals.
For instance, iron, a quintessential transition metal, has a variable valence of +2 or +3. This versatility arises from its d-orbital configuration, which can accommodate two or three unpaired electrons. The valence electrons in these d-orbitals determine its oxidation state and enable iron to participate in a wide range of chemical reactions.
Unveiling the mysteries of transition metals requires a deep understanding of valence electrons. These electrons serve as the bridge between electron configuration, orbitals, and the chemical behavior of these fascinating elements. Their variable valence unlocks a vast realm of possibilities, making transition metals essential components in countless technological advancements.
Applications and Implications: Exploring the Practical Side
The versatility of transition metals stems from their variable valence electron counts. This unique property allows them to form diverse compounds with a wide range of applications. One crucial application is in the field of catalysis, where transition metals serve as catalysts to facilitate chemical reactions. They enable these reactions to occur at significantly faster rates and under milder conditions.
In materials science, transition metals play a vital role in the development of alloys, which are mixtures of metals with enhanced properties. By combining transition metals with other elements, scientists can create materials with tailored properties such as improved strength, durability, and corrosion resistance. These alloys find applications in various industries, including aerospace, automotive, and construction.
Transition metals also hold significant importance in electronics. The ability of transition metals to exist in multiple oxidation states makes them suitable for use as electrodes in batteries and fuel cells. Moreover, their magnetic properties are exploited in the development of electronic devices such as superconductors and magnetic resonance imaging (MRI) scanners.
In this blog post, we have explored the fascinating world of transition metals, delving into their unique properties and the role of valence electrons in shaping their chemical behavior. We have seen how these metals form the foundation of the d-block elements and exhibit remarkable versatility due to their variable valence electron counts.
Throughout this journey, we have gained a deeper understanding of the interconnectedness of d-block elements, transition metals, valence electrons, and their properties. This knowledge provides a solid foundation for further exploration of these essential elements and their far-reaching applications in various fields.