Unveiling The Unstoppable Speed Of Light: Exploring The Cornerstone Of Relativity
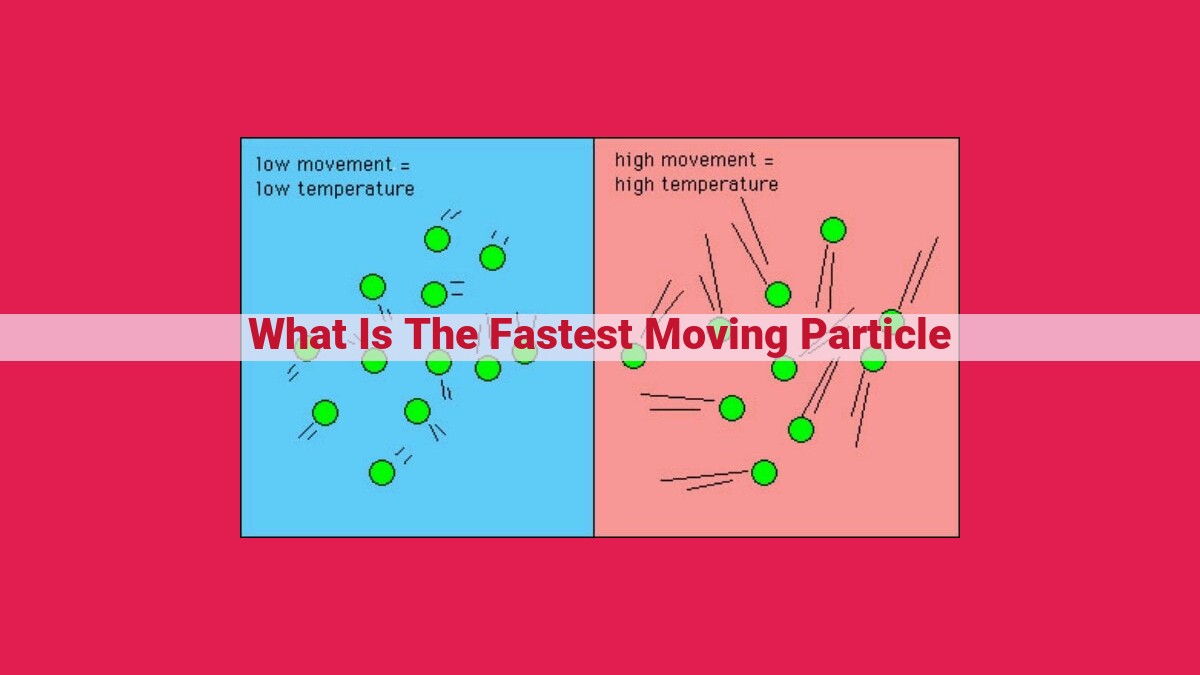
The fastest moving particle is light, traveling at an astounding speed of 299,792,458 meters per second. This speed is a constant in the universe and forms the foundation of Einstein’s theory of relativity, which explains the connection between space, time, and gravity.
The Speed of Light: The Universe’s Ultimate Tracer
Embark on a thrilling journey to the frontiers of physics as we explore the remarkable speed of light, a cosmic constant that governs the very fabric of our existence. Measured at an astounding 299,792,458 meters per second in a vacuum, the speed of light is not just a number but a profound concept that has revolutionized our understanding of the universe.
This elusive speed is intimately intertwined with the realm of electromagnetism—the force that governs interactions between charged particles. Imagine, if you will, a symphony of photons, massless particles of electromagnetic radiation, racing through the void at this incredible pace. This interplay between light and electromagnetism has led to the birth of groundbreaking technologies like lasers, which harness the focused power of light for precise applications in fields ranging from medicine to manufacturing.
Delving deeper into the realm of physics, we find that the speed of light is also central to the theory of relativity. Proposed by the brilliant Albert Einstein, this theory shattered our Newtonian notions of time and space, introducing the concepts of time dilation and length contraction. In the realm of relativity, the speed of light represents an unbreakable barrier, setting the cosmic speed limit for all matter and energy in the universe.
Illuminating the World of Elementary Particles
In the realm of science, where the unfathomably small meets the infinitely grand, we journey into the enigmatic realm of elementary particles. These fundamental building blocks of matter, smaller than atoms and their constituent protons, neutrons, and electrons, hold the secrets to understanding the very fabric of our universe.
Composition and Characteristics of Elementary Particles
The Standard Model of particle physics provides a framework for understanding the diverse array of elementary particles. These particles are classified into two main groups: quarks and leptons. Quarks, the “bricks” of matter, combine in groups of three to form hadrons, including protons and neutrons. Leptons, on the other hand, are “free” particles, such as electrons and neutrinos.
Each particle has a unique set of properties, including charge, spin, and mass. Charge determines the particle’s electromagnetic behavior, while spin describes its intrinsic angular momentum. Mass, a fundamental property, influences the particle’s interactions and behavior.
The Standard Model and Quantum Mechanics
The Standard Model, along with the principles of quantum mechanics, provides a comprehensive explanation of particle interactions. Quantum mechanics describes the wave-particle duality of elementary particles, allowing them to exhibit both particle-like and wave-like characteristics simultaneously.
The Standard Model postulates the existence of force-carrying particles called bosons, which mediate the fundamental forces of nature. These include the photon (electromagnetism), gluon (strong force), and the W and Z bosons (weak force). The interaction of these particles with elementary particles governs the behavior and structure of matter.
High-Energy Physics Research and Particle Accelerators
To unravel the secrets of elementary particles, scientists conduct high-energy physics research using massive particle accelerators. These accelerators, such as the Large Hadron Collider (LHC), propel particles to near-light speeds and collide them with each other.
By studying the resulting particle interactions, physicists gain insights into the nature of fundamental forces, the properties of particles, and the structure of the universe at its most fundamental level. These investigations have led to groundbreaking discoveries, including the confirmation of the Higgs boson, which plays a crucial role in particle mass generation.
The exploration of elementary particles has illuminated our understanding of the universe, revealing the intricate workings of matter and energy. From the Standard Model to the latest discoveries at particle accelerators, scientists continue to push the boundaries of knowledge, unveiling the secrets that lie at the very heart of existence.
Tachyons: Beyond the Speed of Light (Hypothetical)
- Discuss the properties of tachyons and their hypothetical nature
- Explore the implications for faster-than-light travel and special relativity
- Outline current scientific understanding and ongoing research
Tachyons: Beyond the Speed of Light
In the realm of physics, where the laws of nature govern the universe, one elusive concept that has captivated scientists is that of tachyons. These hypothetical particles are rumored to possess the power to transcend the speed of light.
Properties of Tachyons
Tachyons are theorized to be faster-than-light particles, defying the fundamental tenets of special relativity. This extraordinary ability stems from their imaginary mass, a concept that distinguishes them from ordinary particles. Unlike particles with positive mass, which require energy to accelerate, tachyons are postulated to slow down as they gain energy.
Implications for Faster-than-Light Travel
If tachyons do exist, their implications for the world of physics would be profound. They could potentially shatter the established laws of relativity, allowing for faster-than-light communication and travel. Such breakthroughs would revolutionize our understanding of the universe and open up new horizons in space exploration.
Current Understanding and Ongoing Research
While the existence of tachyons remains speculative, scientists continue to explore the possibilities they present. High-energy physics experiments, such as those conducted at the Large Hadron Collider, aim to detect signs of these elusive particles. Researchers also delve into the theoretical implications of tachyons, seeking to reconcile their existence with the accepted laws of physics.
The Elusive Nature of Tachyons
Despite the ongoing research, tachyons remain elusive, their properties and behavior still shrouded in mystery. The very concept of imaginary mass and faster-than-light travel challenges our current understanding of the universe. But as scientists relentlessly pursue the truth, the possibility that tachyons exist may someday be revealed, unlocking new insights into the fundamental nature of reality.
Elusive Neutrinos: Subatomic Ghosts
In the heart of the atomic realm, where particles dance and interact at the most fundamental level, there exist enigmatic entities known as neutrinos. These subatomic ghosts are elusive and intangible, leaving behind only traces of their existence.
Neutrinos are massless particles that carry no electrical charge. They come in three flavors: electron, muon, and tau. These flavors arise from their association with different charged leptons (electrons, muons, and taus).
Weak interactions are the key to understanding neutrinos. These interactions are responsible for nuclear decay, the process that transforms one atomic nucleus into another. Neutrino oscillations, a peculiar phenomenon, occur as neutrinos travel through space and time. During oscillation, neutrinos change flavors, baffling scientists and challenging our understanding of the subatomic world.
Detecting neutrinos is an extraordinary feat. They are notoriously difficult to trap, as they can pass through vast amounts of matter without leaving a trace. However, ingenious detection techniques, such as large underground tanks and sensitive detectors, have unlocked the secrets of these subatomic ghosts.
Neutrinos play a crucial role in astrophysical phenomena. They are produced in immense quantities during nuclear reactions within stars, including our own Sun. Studying neutrinos can shed light on stellar evolution, supernovae, and the enigmatic processes that occur within the depths of the cosmos.
Their unique properties make neutrinos a fascinating subject for ongoing research. Neutrino observatories around the world are dedicated to unraveling the mysteries of these elusive particles. Their findings have the potential to revolutionize our understanding of the subatomic realm and the fundamental nature of the universe itself.
From the Depths of Space: Cosmic Rays
- Describe the origin and composition of cosmic rays
- Explain the properties of high-energy particles and their interactions
- Explore their impact on astrophysics and space science
From the Depths of Space: Cosmic Rays
In the vast expanse of the cosmos, beyond our solar system and beyond the reach of visible light, lies a mysterious and fascinating phenomenon known as cosmic rays. These enigmatic particles, charged with extraordinary energy, bombard our planet from every direction, penetrating the atmosphere in an unceasing stream.
Cosmic rays are not ordinary particles like those in the air we breathe or the soil we tread upon. They are fragments of matter from distant galactic sources, hurtling towards the Earth at velocities approaching the speed of light. Their origins are shrouded in mystery, ranging from supernova explosions to the cataclysmic collisions of neutron stars or black holes.
Upon entering the Earth’s atmosphere, cosmic rays interact with molecules, creating a cascade of secondary particles. This cosmic ballet produces a shower of radiation that can be detected by ground-based observatories using instruments like scintillators and cloud chambers. By studying these traces, scientists seek to uncover the secrets of cosmic rays’ sources, their composition, and their interactions.
The properties of cosmic rays hold profound implications for astrophysics and space science. Their high energies provide a unique probe into the violent processes occurring in the cosmos, deepening our understanding of stellar evolution, black holes, and the formation of heavy elements. Moreover, cosmic rays have a direct impact on the Earth’s atmosphere and climate, and may even play a role in seeding the formation of clouds.
The study of cosmic rays is an ongoing endeavor, with scientists continuously pushing the limits of our knowledge through advanced experiments and innovative technologies. By unraveling the mysteries of these enigmatic particles, we gain a deeper appreciation for the immensity and complexity of the universe we inhabit. And as we venture further into the cosmos, cosmic rays will continue to guide our understanding of the cosmic phenomena that shape our world.
Ripples in Spacetime: Gravitational Waves
In the vast tapestry of the cosmos, space and time are intricately intertwined, woven together by the enigmatic force of gravity. Just as a pebble dropped into a tranquil pond sends ripples spreading outward, so too do massive cosmic events create disturbances in the fabric of spacetime, known as gravitational waves.
Gravitational waves are predicted by Albert Einstein’s groundbreaking theory of general relativity, which revolutionized our understanding of gravity. According to this theory, gravity is not a force that acts instantaneously, but rather a curvature in spacetime caused by the mass and energy of objects. When an object moves or interacts with another object, it creates ripples in spacetime that propagate outward at the astonishing speed of light.
These cosmic ripples, known as gravitational waves, carry valuable information about the cataclysmic events that produce them. One of the most significant sources of gravitational waves is the merger of black holes or neutron stars. When these massive objects collide, they release an enormous amount of energy, creating powerful gravitational waves that ripple through spacetime.
The detection of gravitational waves has opened up a new window into the universe, allowing scientists to observe and study some of the most extreme events that occur in the cosmos. The Laser Interferometer Gravitational-Wave Observatory (LIGO), a pair of highly sensitive detectors located in the United States, made the first direct detection of gravitational waves in 2015, confirming Einstein’s theory and revolutionizing our understanding of gravity.
The study of gravitational waves is still in its infancy, but it has already provided invaluable insights into the nature of black holes, neutron stars, and the universe itself. By listening to the whispers of spacetime, scientists are unraveling the secrets of the cosmos and revealing the hidden wonders that lie beyond our immediate grasp.