Unveiling Phosphate Charge: Its Impact On Biological Processes
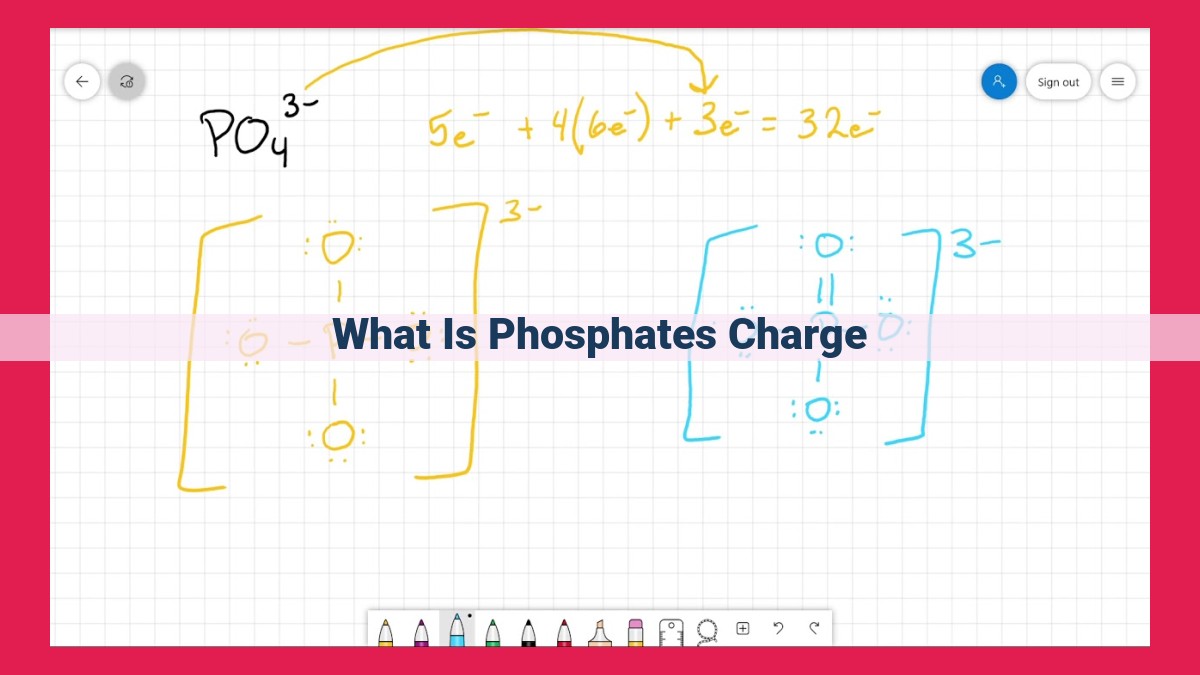
Phosphate charge refers to the electric charge carried by phosphate ions, which are essential components of biological molecules like DNA and RNA. The charge of phosphates depends on the ionization state of the phosphate group, which is influenced by the pH of the environment. In acidic conditions, phosphate groups are mostly protonated and carry a negative charge, while in basic conditions, they deprotonate and become neutral. The charge of phosphates affects their interactions with other molecules and their role in various biological processes, such as energy transfer and signal transduction.
- Define phosphate charge and explain its importance in biological processes.
What is Phosphate Charge? A Guide to Its Importance in Biological Processes
In the intricate dance of biological chemistry, phosphate charge plays a pivotal role. Phosphate, an inorganic anion, carries a negative charge of -3 and exists in various forms, each with its unique charge properties. Understanding these charges is crucial for comprehending a wide range of biological processes.
Phosphate Charge and Biological Processes
Phosphate charge is a key determinant of its reactivity. Anions, including phosphate, are attracted to positively charged ions, forming strong electrostatic bonds. This ability to bind and donate electrons makes phosphate essential for energy transfer in cells. Phosphate is a major component of ATP (adenosine triphosphate), the universal energy currency of cells. ATP acts as a rechargeable battery, providing energy for cellular processes by transferring its phosphate groups to other molecules.
Moreover, phosphate charge influences the structure and function of nucleic acids (DNA and RNA). Phosphate groups form the backbone of nucleic acids, contributing to their stability and charge distribution. Changes in phosphate charge can affect the interactions between nucleic acids and proteins, influencing gene expression and genetic regulation.
Ionization and Phosphate Charge
Phosphate ions exist in different states of ionization, depending on the pH of their environment. At neutral pH, phosphate is predominantly present as a highly charged triprotic anion (H3PO4-). However, in acidic conditions, phosphate can lose protons, becoming a diprotic anion (H2PO4-) or a monoprotic anion (HPO42-).
The pH at which an acid loses half of its protons is called the pKa. Phosphate has three pKa values, corresponding to the loss of each proton. When the pH is below the pKa, the phosphate group is predominantly protonated and carries a positive charge. As the pH increases, the phosphate group deprotonates and gains a negative charge.
Phosphate Buffer: Maintaining pH Balance
In biological systems, pH must be tightly regulated to maintain cellular homeostasis. Phosphate buffers play a crucial role in this regulation. A buffer is a solution that resists changes in pH when small amounts of acid or base are added.
Phosphate buffers contain a mixture of weak acids and weak bases that can donate or accept protons, respectively. The dihydrogen phosphate ion (H2PO4-) and the hydrogen phosphate ion (HPO42-) form a buffer system that can neutralize both acids and bases.
When an acid is added, the H2PO4- ion can donate a proton to form HPO42- ion, neutralizing the acid. Conversely, when a base is added, the HPO42- ion can accept a proton to form H2PO4- ion, neutralizing the base.
Understanding phosphate charge is essential for comprehending its crucial role in biological processes. From energy metabolism to genetic regulation, phosphate charge dictates the interactions and functionality of molecules within cells. By controlling pH through phosphate buffering, cells ensure a stable environment for these essential biochemical reactions.
Ionization and Phosphate Charge
Phosphate charge plays a crucial role in various biological processes. To understand this charge, we need to explore the concept of ionization. Ionization is the process by which an atom or molecule gains or loses electrons, resulting in a change in its charge.
Phosphate is a polyatomic anion with the chemical formula PO4 3-. It can exist in various forms depending on the pH of its environment. At neutral pH, phosphate exists primarily as a fully ionized species, with a net charge of -3. However, as the pH decreases (becomes more acidic), phosphate undergoes a stepwise ionization process, losing protons (H+ ions) and gaining a positive charge.
The ionization of phosphate is influenced by its pKa values. pKa is the pH at which half of the molecules in a solution are ionized. The pKa values for phosphoric acid, the parent acid of phosphate, are:
- pKa1 = 2.15
- pKa2 = 7.20
- pKa3 = 12.35
At pH values below pKa1, phosphate exists predominantly as the fully protonated species, H3PO4. As the pH increases above pKa1, phosphate begins to lose protons and becomes partially ionized, forming H2PO4-. At pH values between pKa1 and pKa2, the majority of phosphate exists as the dihydrogen phosphate ion, H2PO4-.
When the pH rises above pKa2, phosphate continues to lose protons, forming HPO42-. In the physiological pH range of 7.2-7.4, the predominant form of phosphate is HPO42-. Finally, at pH values above pKa3, phosphate loses its final proton and becomes fully ionized as PO4 3-.
Phosphate Buffers
Buffers are solutions that resist changes in pH upon the addition of acid or base. Phosphate ions play a vital role in biological buffer systems, particularly in maintaining the pH of bodily fluids such as blood and intracellular fluids. Phosphate buffers work by absorbing or releasing protons, depending on the pH of the solution.
At acidic pH, phosphate buffers absorb protons, converting H2PO4- to H3PO4. This helps to neutralize the added acid and prevent a significant drop in pH. Conversely, at alkaline pH, phosphate buffers release protons, converting HPO42- to H2PO4-. This helps to neutralize the added base and prevent a significant increase in pH.
The buffer capacity of a solution is a measure of its ability to resist pH changes. Phosphate buffers have a high buffer capacity in the physiological pH range, making them well-suited for maintaining stable pH conditions within biological systems.
The Phosphate Group and Its Charge
In the realm of biological processes, the phosphate group plays a pivotal role. Its interactions with various molecules and ions are crucial for maintaining life’s delicate balance. Understanding the structure and charge of the phosphate group is essential to comprehending its significance.
The phosphate group, denoted as PO₄³⁻, is a polyatomic ion composed of one phosphorus atom and four oxygen atoms. The phosphorus atom forms covalent bonds with each oxygen atom, resulting in a tetrahedral structure. This unique geometry, with its four oxygen atoms surrounding the central phosphorus atom, imparts distinct properties to the phosphate group.
The phosphate group is classified into two forms based on its hydration state: hydrated phosphate and anhydrous phosphate. Hydrated phosphate (HPO₄²⁻) has one water molecule attached to the phosphorus atom, while anhydrous phosphate (PO₄³⁻) has no attached water molecule. These forms interconvert depending on the environment, with hydrated phosphate predominating in aqueous solutions and anhydrous phosphate in nonpolar environments.
The phosphate group’s charge is a critical aspect of its functionality. The phosphate group has a negative charge of -3 due to the presence of four oxygen atoms, which possess higher electronegativity than phosphorus. This negative charge enables the phosphate group to interact with positively charged molecules and ions, such as calcium ions (Ca²⁺), forming stable complexes.
The phosphate group’s ability to ionize depends on the pH of the solution. In acidic conditions, the phosphate group protonates, losing hydrogen ions to form the dihydrogen phosphate ion (H₂PO₄⁻), which has a negative charge of -1. As the pH increases, the dihydrogen phosphate ion further deprotonates to form the hydrogen phosphate ion (HPO₄²⁻), which has a negative charge of -2. Finally, in basic conditions, the hydrogen phosphate ion loses another proton to form the phosphate ion (PO₄³⁻), which has a negative charge of -3.
The different ionization states of the phosphate group allow it to participate in various biological processes, such as energy metabolism, nucleic acid synthesis, and signal transduction. By altering the pH of its environment, the cell can control the ionization state of phosphate groups, fine-tuning their interactions and regulating cellular processes with remarkable precision.
pH and Phosphate Charge: A Tale of Influence
The pH of a solution plays a crucial role in the ionization of phosphate groups, a fundamental aspect of phosphate charge. As pH varies, the ionization state of phosphate ions shifts, leading to a range of charges. This dynamic is of utmost importance in various biological processes.
At a low pH, the phosphate group tends to remain largely protonated, resulting in a positive charge. As the pH increases, the protonation decreases, and the phosphate group begins to lose protons, gradually acquiring a net negative charge.
This ionization process is characterized by a specific pKa, or acid dissociation constant, unique to each phosphate group. When the pH of the solution matches the pKa, the phosphate group exists in a neutral state, with equal protonation and deprotonation.
The pH range at which phosphate ions carry different charges has profound implications in biological systems. For example, in acidic environments (low pH), phosphate ions are predominantly positively charged, allowing them to interact with other negatively charged molecules. Conversely, in basic environments (high pH), phosphate ions are mainly negatively charged, enabling them to bind to positively charged molecules.
Therefore, precise pH control is essential in biological systems involving phosphate, as it directly affects the charge and hence the interactions of phosphate ions. This pH dependency plays a critical role in various cellular processes, such as energy metabolism, nucleic acid synthesis, and protein phosphorylation. Understanding the relationship between pH and phosphate charge is fundamental to comprehending the intricate workings of life’s molecular machinery.
Phosphate Buffer: The Unsung Hero of Biological pH Stability
In the bustling world of biological processes, pH stability reigns supreme. Amidst the myriad molecules that orchestrate this delicate balance, phosphate ions emerge as unsung heroes, playing a crucial role in maintaining a steady internal environment.
The Puzzle of pH
Imagine a pH scale, a spectrum ranging from acidic to basic. Biological systems thrive within a narrow pH window, outside of which their delicate machinery falters. Just as Cinderella’s carriage turns into a pumpkin after midnight, so too can cellular functions unravel when pH deviates excessively from its optimal range.
Phosphate’s Protective Embrace
Enter phosphate ions, nature’s clever buffer. Buffers, like phosphate, have the remarkable ability to resist changes in pH. They operate like pH guardians, neutralizing both acids and bases, ensuring a stable internal environment for biological processes.
How Phosphate Buffers Work: A Tale of Proton Exchange
Phosphate buffers achieve their pH-stabilizing feats through a clever chemical dance. When acids attempt to lower pH, phosphate ions donate protons to neutralize the acidic threat. Conversely, when bases seek to raise pH, phosphate ions absorb protons, effectively lowering the base’s impact.
The Power of Buffer Capacity: Quantifying Phosphate’s Strength
Buffer capacity, a measure of a buffer’s ability to resist pH changes, is crucial in biological systems. Phosphate buffers exhibit high buffer capacity, meaning they can effectively neutralize large amounts of acids or bases without altering pH significantly. This property is vital for maintaining pH stability in environments where pH fluctuations could disrupt cellular functions.
In the intricate symphony of biological processes, phosphate buffers serve as silent protectors, ensuring a stable pH environment essential for life. Their ability to neutralize acids and bases, coupled with their high buffer capacity, makes them indispensable players in maintaining the delicate balance of life’s most fundamental processes. Without phosphate buffers, the pH within living organisms would fluctuate wildly, jeopardizing the very essence of biological systems.