Unlocking The Secrets Of Paralogs: Gene Duplication And Functional Divergence
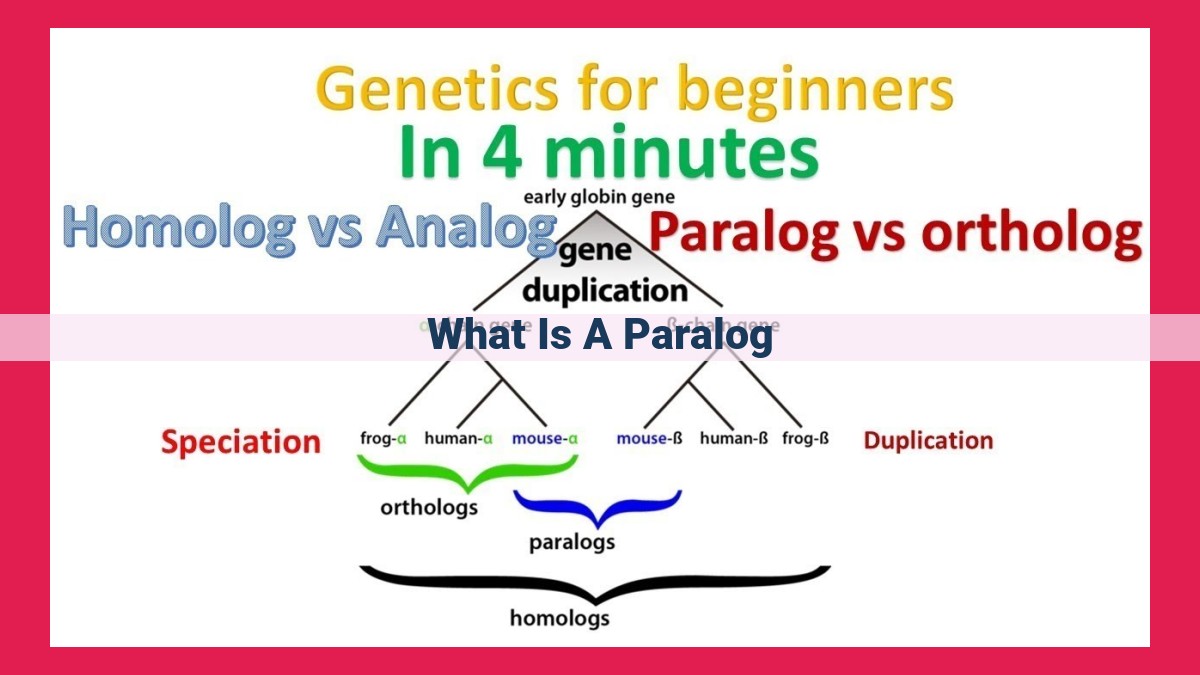
Paralogs arise from gene duplication events, leading to genes with a shared origin but distinct functions. They are common within a genome and can also be found in different species. Paralogs evolve divergently after duplication, with one copy retaining the original function and the other acquiring new roles through the accumulation of mutations. This functional divergence can range from gradual to rapid, resulting in paralogs with similar or entirely different roles. Paralogs serve as evidence of gene duplication and play a crucial role in the diversification of gene function and the evolution of phenotypic complexity.
- Define paralogs as genes with a shared evolutionary origin that have acquired different functions due to gene duplication.
Paralogs: The Evolutionary Duplicates with Distinct Roles
Imagine a world where genes, the blueprints of life, could make copies of themselves. These copies, known as paralogs, are like twins separated at birth, carrying the same genetic heritage but destined for different paths.
Paralogs arise through the fascinating process of gene duplication. Imagine a gene being copied like a Xerox machine. This duplication can happen during cell division or through a process called retrotransposition, where a gene is converted into a mobile copy that can insert itself into other parts of the genome. These duplicated genes are like blank slates, ready to take on new roles and functions.
The Paralog Family: Distinct but Related
Paralogs are closely related to their siblings, known as orthologs. Orthologs are genes that share a common ancestor but have remained largely unchanged in function across different species. Paralogs, on the other hand, have taken on different functions, giving rise to the diversity of life forms we see today.
The Journey to Divergent Evolution
After gene duplication, paralogs embark on a journey of divergent evolution. One copy may maintain the original function, while the other accumulates mutations and embarks on a new evolutionary path. Like siblings taking different career paths, paralogs gradually acquire specialized functions, contributing to the complexity of biological systems.
The Paralog Puzzle: Clustering and Divergence
Paralogs often reside in clusters within the genome, like siblings living in the same neighborhood. These clusters are remnants of tandem duplication events, where multiple copies of a gene are created side by side.
The functional divergence in paralogs can vary greatly. Some may retain similar functions, while others acquire entirely different roles. These diverse outcomes provide the raw material for evolutionary innovation, allowing species to adapt to changing environments and challenges.
Case Study: The Human Hemoglobin Genes
The human hemoglobin genes HBA1 and HBB offer a compelling example of paralog functional specialization. These genes have evolved distinct roles in hemoglobin expression during different life stages. HBA1 is primarily active in adults, while HBB plays a vital role in fetal hemoglobin production.
Paralogs: The Unsung Heroes of Evolution
Paralogs are the unsung heroes of evolutionary biology, providing evidence of gene duplication and the power of genomic diversification. They have played a pivotal role in the evolution of complex biological systems and the diversity of life. Understanding paralogs is crucial for unraveling the genetic mechanisms that have shaped the history of life on Earth.
Unveiling the Mechanisms of Gene Duplication: A Tale of Unequal Crossover and Retrotransposition
In the realm of genetics, gene duplication plays a pivotal role in the evolution of species. It’s a process that duplicates a gene, creating two copies with identical sequences. These duplicated genes, known as paralogs, venture on different evolutionary paths, acquiring distinct functions. But how exactly do genes duplicate?
One intriguing mechanism is unequal crossover during meiosis, the cell division that creates gametes (eggs and sperm). During this intricate dance, chromosomes pair up and exchange genetic material. Sometimes, this exchange goes awry, resulting in one chromosome gaining an extra copy of a gene while the other loses it. This unequal distribution leads to the creation of paralogs.
Another player in gene duplication is retrotransposition. This molecular acrobatics involves the creation of an RNA copy of a gene, which is then reverse-transcribed back into DNA. This newly synthesized DNA can insert itself elsewhere in the genome, creating a duplicate copy of the gene.
Paralogs are widespread within genomes and may also exist in different species. They arise as a result of duplication events that can occur randomly or be driven by evolutionary pressures. These duplicated genes provide genetic raw material for functional divergence, which is the process by which paralogs acquire distinct roles.
The study of gene duplication and the resulting paralogs has shed light on the evolutionary history of species. It’s a story of genetic tinkering, where gene duplications serve as pivotal moments of innovation. These duplicated genes, like budding entrepreneurs, venture into new functional territories, contributing to the diversification of species and the intricate tapestry of life.
Paralogs and Homologs: Exploring the Evolutionary Landscape of Genes
In the intricate tapestry of genetics, gene duplication plays a pivotal role in shaping the diversity and complexity of life. One fascinating product of gene duplication is paralogs, genes that share a common ancestral origin but have diverged in function. To fully grasp the nature of paralogs, it’s essential to understand their relationship with two other key concepts in evolutionary biology: orthologs and homologs.
Orthologs: Guardians of Conserved Function
In the realm of different species, orthologs emerge as genes that perform identical functions. They are the genetic counterparts that have been passed down from a common ancestor without undergoing any significant functional changes. Orthologs provide a window into the evolutionary history of species, serving as crucial reference points for understanding the conservation of essential biological processes.
Homologs: A Tale of Common Ancestry
Homologs, on the other hand, encompass a broader category of genes that share a common origin but may have acquired different functions over time. This divergence can arise from gene duplication events, where one copy retains its ancestral function while the other embarks on a new evolutionary path. Homologs provide insights into the diversification of gene function and the adaptive evolution of species.
Paralogs: The Product of Duplication and Divergence
In contrast to both orthologs and homologs, paralogs originate from gene duplication events within a single genome. These duplicated genes are initially redundant in function but eventually diverge in their roles, a process driven by natural selection. The result is a pair of genes that share a common evolutionary ancestor but have acquired distinct functions.
A Tale of Three Genes: Orthologs, Homologs, and Paralogs
To illustrate the nuanced relationship between these concepts, consider three hypothetical genes: Gene A, Gene B, and Gene C. Gene A and Gene B represent orthologs, as they fulfill the same function in two different species. Gene C, however, is a homolog of both Gene A and Gene B, sharing a common evolutionary ancestor but performing a different function. And finally, Gene A and Gene C are paralogs, as they originated from a gene duplication event within the same genome and have diverged in function.
By understanding the intricate relationships between orthologs, homologs, and paralogs, we gain a deeper appreciation of the dynamic and evolving nature of genes. Gene duplication and divergence have been key drivers in the diversification of life, enabling species to adapt and thrive in a changing environment.
Divergent Evolution of Paralogs: A Tale of Genetic Duplication and Functional Innovation
After the fascinating event of gene duplication, paralogs embark on a remarkable evolutionary journey called divergent evolution. This process transforms these duplicate genes into entities with distinctive functions, often breaking away from their ancestral roles.
One copy of the duplicated gene typically inherits the mantle of its progenitor, faithfully preserving its original function. However, the other copy, free from such constraints, becomes a playground for genetic exploration. Over time, it accumulates mutations that subtly alter its DNA code and, like a sculptor chiseling away at stone, gradually reshape its function.
This divergence can be subtle, with the new paralog assuming a slightly modified version of its original role. In other cases, the transformation is dramatic, as the paralog embarks on an entirely novel path, acquiring a function that bears little resemblance to its ancestor.
This metamorphosis is a testament to the power of natural selection. As paralogs diverge, one copy becomes fine-tuned for a specific task, while the other explores new functional frontiers, expanding the repertoire of the organism. It’s a biological ballet, where each step brings forth a new adaptation, a new layer of complexity in the intricate tapestry of life.
Clustered Paralogs
- Discuss the frequent occurrence of paralogs in clusters within the genome.
- Attribute this clustering to tandem duplication events, where multiple copies of a gene are created adjacent to each other.
Clustered Paralogs
Paralogs, genes sharing a common ancestor but divergent functions, often reside in clusters within the genome. These clusters arise through tandem duplication events, where multiple copies of a gene are duplicated adjacent to one another.
Imagine a group of genes lined up like soldiers on a chromosome. During cell division, a mishap occurs. Instead of each gene being copied and separated correctly, one gene gets duplicated twice, resulting in three identical copies right next to each other. This kind of duplication gives rise to a clustered paralogs.
Clustered paralogs offer a unique perspective into gene evolution. By comparing the sequences of the paralogs within a cluster, researchers can trace the evolutionary changes that have occurred since the gene duplication event. This can provide valuable insights into how gene function has diversified over time.
The clustering of paralogs also facilitates coordinated regulation. Genes located in close proximity on the chromosome are often regulated by the same control elements, ensuring that they are expressed in a coordinated manner. This coordination is particularly important for gene families, where multiple paralogs perform related functions.
For example, genes encoding the hemoglobin subunits in humans are clustered on chromosome 11. This clustering allows for the precise regulation of hemoglobin production, which is critical for oxygen transport throughout the body. During different stages of development, specific paralogs within the cluster are expressed to meet the changing oxygen demands of the organism.
Clustered paralogs are not just a genetic curiosity, they play a vital role in evolutionary biology. They provide evidence of gene duplication, a key mechanism for generating new genetic material and driving evolutionary innovation. By comparing clustered paralogs, scientists can gain insights into the processes that have shaped the diversity of life on Earth.
Functional Divergence in Paralogs: A Tale of Evolutionary Innovation
Paralogs, duplicate genes born from a common ancestor, embark on divergent journeys in the realm of evolution. After gene duplication liberates them from their ancestral shackles, paralogs embark on paths of functional specialization, ranging from gradual shifts to rapid transformations.
Some paralogs maintain close ties to their original role, like siblings who share a legacy but pursue different paths. They retain similar functions, like two siblings who work in related fields. For instance, the alpha- and beta-globin genes, paralogs responsible for producing hemoglobin, continue to collaborate in oxygen transport, albeit with subtle differences.
Others, however, break free from their ancestral ties, embracing bold new roles. Like siblings who venture into uncharted territories, these paralogs acquire entirely different functions. Consider the Hox genes, a family of paralogs that once played a role in body axis formation. Today, they orchestrate a symphony of developmental processes, shaping everything from limbs to facial features.
Gradual Divergence: From Subtle Shifts to Profound Differences
The functional divergence of paralogs can unfold like a story with a slow-burning plot. After gene duplication, one copy undergoes gradual accumulation of mutations, while the other retains the original function. Over eons, these mutations reshape the gene’s sequence and function, gradually distancing it from its paralog.
Rapid Divergence: A Bolt from the Evolutionary Blue
In some cases, paralogs experience a sudden burst of evolutionary innovation. Like a meteor crashing into a lake, a major mutation or recombination event can dramatically alter the gene’s sequence and function. This rapid divergence can lead to paralogs that are completely unrecognizable from their ancestral counterparts, opening up new avenues for evolution.
A Tapestry of Functional Roles: From Housekeeping to Cell Identity
The functional diversity of paralogs is a testament to evolution’s boundless creativity. They perform a multitude of tasks, from housekeeping chores like DNA repair to defining cell identity and regulating development. Some paralogs even transition between roles, like actors playing different characters in a grand evolutionary play.
Paralogs stand as key players in the evolutionary saga, their functional divergence driving the diversification and complexity of life forms. From subtle shifts to dramatic transformations, their stories unveil the power of gene duplication and the endless potential for evolutionary innovation.
Case Study: Human Hemoglobin Genes, Unraveling the Functional Specialization of Paralogs
Meet the Hemoglobin Team: HBA1 and HBB
Hemoglobin, the oxygen-carrying superhero in our red blood cells, is assembled from two types of polypeptide chains: alpha and beta. These chains are encoded by two paralogous genes: HBA1 and HBB.
A Tale of Duplication and Divergence
HBA1 and HBB originated from a common ancestral gene through gene duplication, an evolutionary dance that creates copies of genes. Over time, these paralogs embarked on divergent paths, accumulating mutations and adapting to distinct roles in hemoglobin production.
Dynamic Duo: Stage-Specific Expression
HBA1 and HBB have evolved to exhibit stage-specific expression during our development. During fetal life, HBA1 takes the lead, producing alpha chains for the embryonic and fetal hemoglobins. As we emerge from the womb, HBB steps into the spotlight, cranking out alpha chains for adult hemoglobin, the workhorse of oxygen transport in our bodies.
Functional Fine-tuning: A Symphony of Hemoglobins
The different hemoglobins produced by HBA1 and HBB are tailored to the unique needs of each developmental stage. Embryonic and fetal hemoglobins have a higher affinity for oxygen, ensuring an adequate supply to the growing fetus. Adult hemoglobin, on the other hand, is precisely tuned to transport oxygen throughout our bodies as adults.
Paralogs: The Engine of Evolutionary Innovation
The story of HBA1 and HBB showcases the power of paralogs in evolutionary biology. Gene duplication provides raw material for functional innovation, allowing organisms to adapt to changing environments and expand their phenotypic complexity. Without the divergence of these paralogs, we would lack the stage-specific control of hemoglobin expression that is essential for our survival and development.
Paralogs: Key Players in Evolutionary Innovation
In the fascinating world of genetics, paralogs stand out as key characters driving evolutionary change. These genes share a common ancestor but have embarked on unique paths, acquiring distinct functions that have shaped the diversity of life on Earth.
Duplicating Success: The Genesis of Paralogs
Paralogs arise through the process of gene duplication. Imagine a cosmic event where a gene makes a copy of itself, creating an identical twin. Over time, the twins drift apart, accumulating changes and developing their own identities.
Orthologs versus Paralogs: A Tale of Two Cousins
In the genetic family tree, orthologs are cousins who share a common ancestor and retain similar functions in different species. Homologs, on the other hand, are distant relatives with a shared evolutionary history but may have divergent functions. Paralogs, however, are those special cousins who share a recent ancestor and have acquired distinct identities within the same species.
Unleashing Divergence: Paralogs Chart Their Own Course
After duplication, paralogs embark on divergent evolutionary paths. One may stick to the traditional family role, while its adventurous sibling explores uncharted territories. This functional divergence can range from subtle tweaks to radical transformations, creating a symphony of genetic diversity.
From Clusters to Complexity: Paralogs as Evolutionary Architects
Paralogs often cluster together in the genome, forming genetic neighborhoods. These clusters are relics of tandem duplication events, where genes multiply like rabbits. This localized gene expansion can fuel rapid evolutionary innovation, giving rise to new traits and increasing the phenotypic complexity of species.
A Case in Point: The Hemoglobin Saga
The human hemoglobin genes (HBA1 and HBB) exemplify the power of paralogs. These cousins have specialized in different roles, with HBA1 producing hemoglobin in adults and HBB taking the lead during fetal development. This division of labor underscores the functional diversification enabled by paralogs.
Significance in Evolutionary Biology: Paralogs’ Evolutionary Impact
Paralogs are not just genetic oddities; they are the catalysts for evolutionary change. They provide evidence of gene duplication, a fundamental force shaping the diversity of life. By diversifying gene function, paralogs have fueled the evolutionary innovation that has transformed the Earth’s inhabitants.