Demystifying Neurons: Unlocking The Essential Building Blocks Of The Nervous System
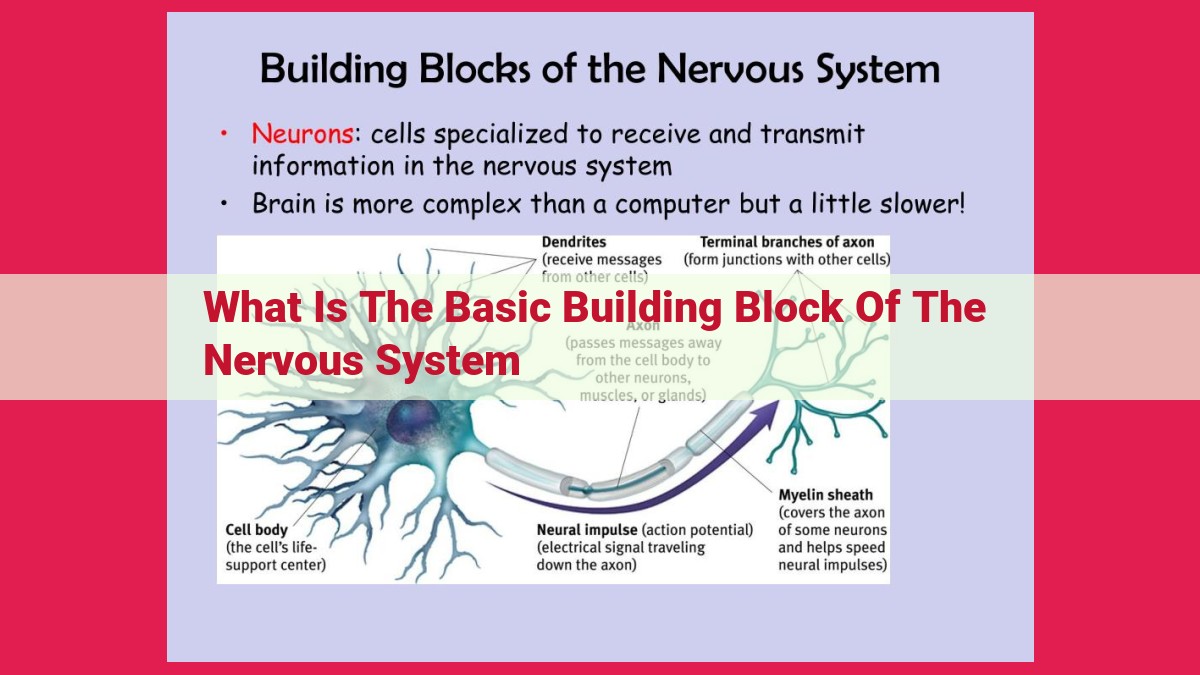
Neurons, the basic building blocks of the nervous system, are specialized cells responsible for receiving, processing, and transmitting electrical and chemical signals. Composed of a cell body, dendrites, axon, and axon terminal, neurons receive signals through dendrites, integrate them in the cell body, and transmit signals via the axon to the axon terminal, where neurotransmitters are released to facilitate communication with other neurons or target cells. This intricate network of neurons enables the complex functions of the nervous system, including sensation, movement, cognition, and emotion.
Journey into the Marvelous World of Neurons: The Building Blocks of Our Nervous System
In the intricate tapestry of life, the nervous system stands as a masterpiece of biological engineering, orchestrating our every thought, action, and sensation. At its core lies a remarkable network of specialized cells called neurons, which serve as the fundamental building blocks of this vital system.
Imagine a vast city teeming with countless citizens, each performing a specific role to ensure the metropolis thrives. The nervous system is much like this urban labyrinth, neurons acting as the bustling individuals responsible for the system’s seamless operation. Their intricate interactions form the foundation of our very existence, enabling us to navigate the world around us.
Neurons: The Cornerstone of Neural Communication
In the intricate realm of the human body, a vast network of specialized cells orchestrates every thought, movement, and sensation. These cells, known as neurons, serve as the fundamental building blocks of the nervous system, enabling us to perceive, process, and respond to the world around us.
The Anatomy of a Neuron: A Structural Marvel
Each neuron resembles a microscopic tree, with an intricate architecture that reflects its unique functions. A central cell body contains the nucleus and other essential organelles that govern the neuron’s metabolism and genetic machinery.
From the cell body sprout numerous branches called dendrites, which act as signal receivers, collecting information from neighboring neurons. These branches increase the neuron’s surface area, enhancing its ability to gather incoming signals.
Extending from the cell body is the axon, a remarkable extension that transmits signals to other neurons. Like a high-speed highway, the axon conveys electrical impulses over long distances, connecting neurons across the vast expanse of the nervous system.
At the end of the axon lies the axon terminal, a specialized structure where signals are transferred to other neurons. These axon terminals house vesicles filled with neurotransmitters, chemical messengers that facilitate communication between neurons.
Dendrites: Receiving Signals
- Discuss the role of dendrites in receiving signals from other neurons.
- Explain how multiple dendrites increase the neuron’s surface area for signal reception.
Dendrites: The Signal Receptionists of the Nervous System
In the intricate tapestry of the nervous system, dendrites emerge as the gatekeepers of neuronal communication. These delicate, tree-like extensions branch out from the neuron’s cell body like a vast network, reaching outward to connect with other neurons. Their primary mission? To receive and collect incoming signals, forming the first step in the intricate symphony of neural transmission.
Increased Surface Area for Enhanced Signal Reception
Imagine a neuron as a tiny city, with dendrites acting as its bustling streets. The more streets there are, the easier it becomes for citizens (signals) to enter and navigate. Similarly, the greater the number of dendrites a neuron possesses, the larger its surface area for receiving signals.
This increased surface area allows neurons to gather signals from multiple sources simultaneously. Think of it as having several antennas instead of just one, maximizing the potential for signal reception. As a result, neurons can integrate a wide range of inputs and make more informed decisions about whether to generate an action potential.
How Dendrites Function
Dendrites are equipped with specialized receptors that bind to neurotransmitters released from neighboring neurons. When a neurotransmitter attaches to a receptor, it triggers a chemical reaction that generates a small electrical signal called a graded potential. This graded potential travels along the dendrite towards the cell body, diminishing in strength as it goes.
If the graded potential reaches a certain threshold at the cell body, it triggers the generation of an action potential. This action potential, an all-or-nothing electrical impulse, then travels down the axon, initiating the next stage of neuronal communication.
Dendrites play a crucial role in the nervous system’s ability to receive, process, and transmit information. Their extensive branching and vast surface area allow neurons to collect and integrate signals from multiple sources, ensuring efficient and effective communication throughout the nervous system. Without these signal receptionists, our brains and bodies would be unable to function as the complex, coordinated systems that they are.
Cell Body: The Command Center of Neurons
Nestled at the heart of every neuron lies the cell body, the neuron’s metabolic powerhouse and command center. This bustling hub orchestrates the neuron’s life-sustaining functions, ensuring it can receive, process, and send signals with lightning-fast precision.
Within the cell body, a symphony of metabolic reactions unfolds, fueling the neuron’s energy needs. Mitochondria, the tiny powerhouses of the cell, generate adenosine triphosphate (ATP), the neuron’s primary energy currency. This energy sustains a myriad of essential processes, from maintaining ion gradients to synthesizing neurotransmitters.
Equally vital is the cell body’s role as an integrator of signals. Like a meticulous conductor, it gathers electrical impulses from dendrites, the neuron’s signal-receiving antennae. A flurry of ion channels, embedded in the cell body’s membrane, meticulously monitor the incoming signals. If the combined electrical charge reaches a critical threshold, known as the threshold potential, the neuron fires an action potential, an electrical impulse that races down the neuron’s axon, transmitting the signal to its next destination.
The Axon: Nature’s Telegraph
Imagine your nervous system as a vast network of communication lines, spanning the length and breadth of your body. These lines are the axons, and they play a crucial role in transmitting signals from the cell body to the axon terminal.
Think of the axon as a long, slender extension of the neuron, acting like a telegraph wire. These wires carry electrical impulses from the neuron’s cell body, where they integrate incoming signals, to the axon terminal.
Myelination: Speeding Up the Telegraph
Now, let’s talk about a special feature of axons: myelination. Imagine insulation wrapped around an electrical wire to prevent signal loss. Myelin, a fatty substance, does the same for axons. It insulates them, allowing electrical impulses to travel faster and more efficiently.
The Powerhouse of Signal Transmission
The axon’s main function is to conduct these electrical impulses, carrying them from the cell body to the axon terminal, where they will trigger the release of neurotransmitters. Like a telegraph transmitting messages, the axon ensures that signals reach their destination quickly and effectively.
Axons are the backbone of neural communication, enabling the rapid and efficient transmission of signals throughout the nervous system. Their myelination allows for even faster signal transmission, just as sophisticated insulation improves the speed of data cables. These hardworking axons are the unsung heroes behind the coordination and functionality of our bodies and minds.
Axon Terminal: The Communication Hub of Neurons
At the end of each neuron, there lies a specialized structure known as the axon terminal. Imagine it as a tiny bulb-like structure responsible for the crucial task of transmitting electrical signals to other neurons across a communication gap called the synaptic cleft.
The axon terminal is packed with neurotransmitters, chemical messengers that carry the electrical signals across this gap. These neurotransmitters are stored in tiny sacs called synaptic vesicles. When an electrical signal reaches the axon terminal, voltage-gated calcium channels open, allowing an influx of calcium ions. This calcium influx triggers the fusion of synaptic vesicles with the terminal membrane, releasing neurotransmitters into the synaptic cleft.
These neurotransmitters then bind to specific receptors on the membrane of the adjacent neuron, triggering a chain reaction that either excites or inhibits the receiving neuron. Excitatory neurotransmitters, such as glutamate, increase the likelihood of the receiving neuron firing an action potential, while inhibitory neurotransmitters, such as GABA, decrease the likelihood.
By releasing neurotransmitters, axon terminals play a vital role in shaping communication within the nervous system. They allow neurons to “talk” to each other, transmitting information about sensory stimuli, thoughts, emotions, and motor commands. Without the axon terminal and its neurotransmitter release mechanism, these electrical signals would never be able to cross the synaptic cleft, and interneuronal communication would be impossible.
Synapse: The Interneuronal Junction
Within the intricate labyrinth of the nervous system, neurons communicate through specialized junctions known as synapses. Synapses serve as communication hubs, facilitating the transmission of electrical and chemical signals between neurons. They allow neurons to share information and coordinate complex bodily functions.
Imagine a synapse as a minuscule bridge connecting two neurons. On one side lies the presynaptic neuron, which sends the signal. On the other side resides the postsynaptic neuron, which receives the signal. The synaptic cleft, a narrow gap between the two neurons, separates them.
When an electrical signal reaches the axon terminal of the presynaptic neuron, it triggers the release of neurotransmitters, chemical messengers that carry the signal across the synaptic cleft. These neurotransmitters bind to receptors located on the postsynaptic neuron’s surface.
The binding of neurotransmitters triggers a chain of events within the postsynaptic neuron. In excitatory synapses, neurotransmitters depolarize the postsynaptic neuron, making it more likely to fire an electrical signal. On the other hand, in inhibitory synapses, neurotransmitters hyperpolarize the postsynaptic neuron, reducing the likelihood of it firing.
The strength of a synapse, known as its synaptic strength, depends on various factors, including the number of neurotransmitter receptors, the amount of neurotransmitter released, and the duration of neurotransmitter binding to receptors. Synaptic strength can change over time, a phenomenon known as synaptic plasticity, which underlies the brain’s ability to learn and adapt.
Synapses are essential for the proper functioning of the nervous system. They enable the coordination of sensory, motor, cognitive, and emotional processes. Dysfunctional synapses can lead to a wide range of neurological disorders, including Alzheimer’s disease and Parkinson’s disease.
Understanding the structure and function of synapses provides a deeper appreciation for the intricate mechanisms that govern our thoughts, actions, and emotions. By delving into the intricacies of these interneuronal junctions, we open up new possibilities for exploring the mysteries of the human mind.
Neurotransmitters: The Chemical Messengers of the Nervous System
Within the bustling metropolis of the nervous system, neurons serve as the tireless messengers, carrying vital information throughout the body. Their ability to communicate effectively relies on the presence of neurotransmitters, the tiny chemical messengers that facilitate signal transmission between nerve cells.
The Symphony of Neurotransmitters
Neurotransmitters are a diverse family of molecules, each with its own distinct role to play in the symphony of brain activity. Glutamate, the most prevalent neurotransmitter, orchestrates the primary excitatory signals that drive neural communication. It’s the spark that ignites the fire of neuronal activity.
Conversely, GABA (gamma-aminobutyric acid) serves as the nervous system’s calming influence. It orchestrates inhibitory signals, dampening excessive neuronal activity and ensuring a harmonious balance in neural communication.
The Mood Regulators
Other neurotransmitters hold sway over our emotions and mental well-being. Dopamine floods us with pleasure and reward, motivating us to seek out desirable experiences. Serotonin bathes us in contentment and tranquility, fostering a sense of calm and well-being.
Epinephrine and norepinephrine ignite our fight-or-flight response, preparing us for action in the face of perceived threats. Acetylcholine orchestrates memory formation, helping us to store and retrieve precious experiences.
The Messenger Molecules
Neurotransmitters are released into the synapse, the narrow gap between neurons. They then bind to specific receptors on the receiving neuron, triggering a cascade of electrical and chemical events that propagate the signal through the nervous system.
It’s remarkable to consider that these tiny molecules orchestrate a vast array of bodily functions, from our heartbeat to our thoughts and emotions. They are the unsung heroes of our nervous system, tirelessly ensuring the efficient and seamless flow of information that governs every aspect of our being.