Unlocking Magnetic Fields: Visualizing And Understanding With Magnetic Field Line Models
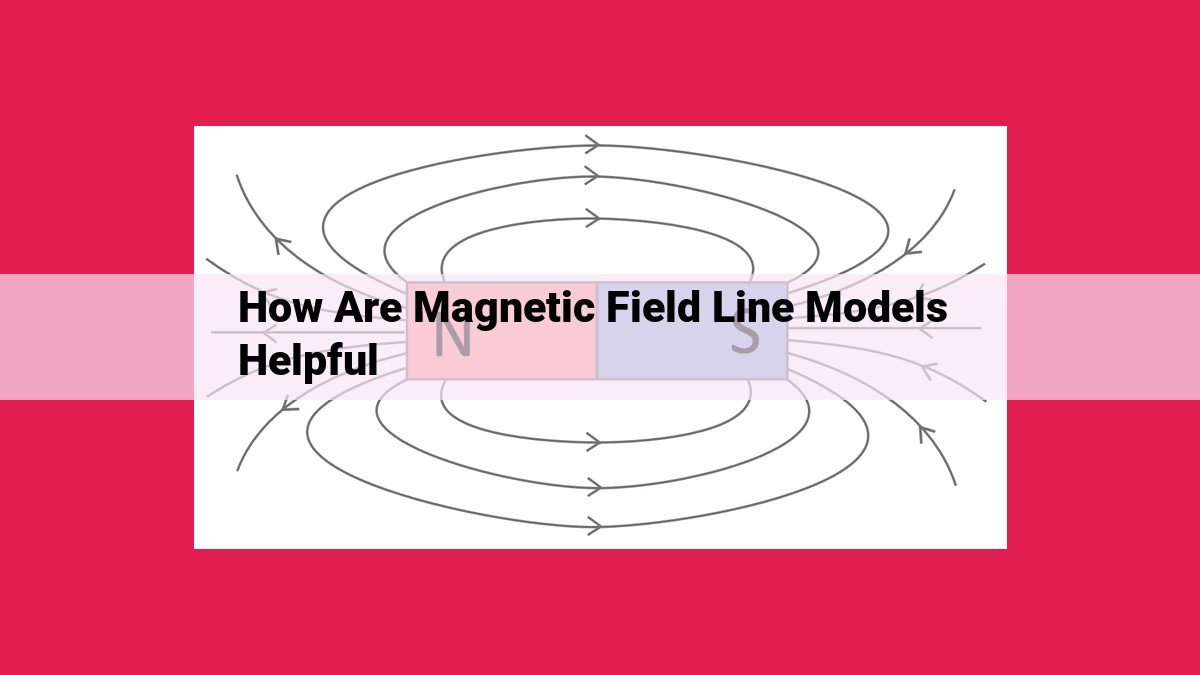
Magnetic field line models provide a visual representation of the direction and strength of magnetic fields. They facilitate understanding of magnetic force interactions, including the Lorentz force, magnetic dipole moment, and magnetic torque. These models aid in the analysis of magnetic circuits, design of magnets and magnetic devices, and prediction of magnetic behavior. They simplify complex magnetic fields using approximations and equivalent circuits, making them valuable for qualitative and quantitative analysis, education, and research.
Visualization of Magnetic Fields:
- Discuss magnetic field lines as a visual representation of direction and strength.
- Explain how to determine magnetic field strength and calculate magnetic flux.
Visualizing Magnetic Fields: Unveiling the Invisible Force
Magnetic fields, the invisible forces that surround magnets and current-carrying conductors, play a crucial role in our everyday lives. From the motors in our appliances to the Earth’s protective magnetic shield, understanding magnetic fields is essential for navigating the world around us.
Magnetic Field Lines: Mapping the Invisible
Imagine magnetic fields as invisible fields of force that flow out from the north pole of a magnet and return to the south pole. Magnetic field lines are a visual representation of these fields, providing a roadmap of their direction and strength. The closer the field lines are together, the stronger the magnetic field.
Measuring Magnetic Strength: A Question of Gauss
The strength of a magnetic field is measured in units of Gauss (G). The higher the Gauss value, the stronger the magnetic field. The magnetic field strength decreases as you move away from the magnet or current-carrying conductor.
Calculating Magnetic Flux: Quantifying the Flow
Magnetic flux is the amount of magnetic field that passes through a given area. It is calculated as the magnetic field strength multiplied by the area perpendicular to the field lines. Magnetic flux is an important quantity in many applications, such as designing transformers and motors.
Understanding Magnetic Force Interactions: The Dance of Charged Particles
When you think of magnets, you might imagine their ability to attract or repel each other. But beneath this simple interaction lies a fascinating world of force and motion: the interplay between moving charged particles and magnetic fields.
The Lorentz Force: A Guiding Hand
Imagine a charged particle like an electron zipping through a magnetic field. It experiences an invisible force known as the Lorentz force, which steers its path like an unseen conductor. This force depends on the particle’s charge (positive or negative), velocity, and the strength of the magnetic field.
Delving into Magnetic Dipole Moments
Some particles, like electrons themselves, behave like tiny magnets with their own magnetic dipole moments. This moment measures their strength and orientation. When such particles encounter a magnetic field, they tend to align themselves with it.
Magnetic Torque: A Twisting Effect
This alignment doesn’t happen instantaneously but involves a twisting force called magnetic torque. Imagine a compass needle freely spinning in a magnetic field. Magnetic torque gradually rotates it until it points along the field lines, like a dancer responding to a choreographer’s cues.
Beyond the Basics: Applications Galore
Magnetic force interactions play a vital role in many technological marvels we use daily. They form the basis for:
- Electric motors and generators that power our devices and light up our lives
- Magnetic resonance imaging (MRI) scans that peer inside our bodies, revolutionizing medical diagnosis
Visualizing the Unseen: Simulations and Experiments
Understanding magnetic force interactions can be daunting, but interactive simulations and real-world experiments bring them to life. By observing how charged particles behave in magnetic fields, we gain insights into this fascinating realm of nature’s forces.
Analysis of Magnetic Circuits:
- Introduce magnetic reluctance as a measure of resistance to magnetic flux flow.
- Determine magnetic flux density and apply Ampère’s circuital law to study current-carrying conductors and magnetic fields.
Analysis of Magnetic Circuits: Understanding the Resistance to Magnetic Flux Flow
In the realm of magnetism, understanding magnetic circuits is crucial. Just as electric circuits quantify the flow of electric current, magnetic circuits analyze the flow of magnetic flux.
Magnetic Reluctance: The Resistance to Magnetic Flux
Analogous to electrical resistance in electrical circuits, magnetic circuits also have a measure of resistance, known as magnetic reluctance. Reluctance quantifies the opposition to the flow of magnetic flux in a magnetic circuit. The higher the reluctance, the harder it is for magnetic flux to flow.
Determining Magnetic Flux Density with Ampère’s Law
Magnetic flux density is a measure of the strength of a magnetic field at a given point. Ampère’s circuital law provides a powerful tool for calculating magnetic flux density. By tracing a closed path around a current-carrying conductor, the law relates the current enclosed to the line integral of the magnetic field around the path.
Studying Current-Carrying Conductors and Magnetic Fields
Magnetic circuits provide a framework for analyzing the magnetic fields generated by current-carrying conductors. By considering the magnetic reluctance of different materials and applying Ampère’s law, engineers can predict the distribution of magnetic flux in a given circuit. This knowledge is essential for designing and optimizing electrical devices, such as transformers and motors.
Design of Magnets and Magnetic Devices:
- Explore the properties and uses of permanent magnets.
- Explain how to create electromagnets and their applications.
- Discuss magnetic shielding techniques.
Design of Magnets and Magnetic Devices
The realm of magnetism extends beyond theoretical understanding and into the practical world of real-world applications. Magnets and magnetic devices play an integral role in countless technologies, from our everyday gadgets to medical advancements.
Permanent Magnets
These magnetic marvels retain their magnetic properties without requiring an external power source. Their durable nature makes them ideal for applications where a consistent magnetic field is crucial. From refrigerator magnets to the motors in electric vehicles, permanent magnets have found widespread use.
Electromagnets
Unlike their permanent counterparts, electromagnets derive their magnetism from an electric current. By passing an electric current through a coil of wire, a magnetic field is generated. The strength of this field is directly proportional to the current flowing through the coil. Electromagnets offer versatility as their magnetic field can be easily controlled by adjusting the current. They are commonly employed in MRI scanners, loudspeakers, and industrial lifting equipment.
Magnetic Shielding
In certain applications, it is essential to control or minimize magnetic fields. Magnetic shielding techniques provide a solution by using materials with high magnetic permeability to create a barrier that channels magnetic fields away from sensitive areas. This technique finds application in protecting electronic devices from harmful magnetic interference and in reducing the magnetic field exposure in medical and scientific settings.
SEO-Optimized Version
Discover the Intriguing World of Magnets and Magnetic Devices
Step into the fascinating world of magnetism and uncover the practical applications of magnets and magnetic devices that shape our everyday lives.
Permanent Magnets: The Unsung Heroes of Everyday Tech
These magnetic workhorses, with their long-lasting power, play a vital role in our modern world. From the refrigerator magnets that hold our notes to the motors that propel electric vehicles, permanent magnets work tirelessly behind the scenes.
Electromagnets: The Dynamic Partners of Electricity
Unlike their permanent counterparts, electromagnets rely on electric currents to generate their magnetic fields. This dynamic duo allows for precise control over the magnetic field strength, making them invaluable in MRI scanners, loudspeakers, and the industrial giants responsible for lifting heavy loads.
Magnetic Shielding: A Sanctuary from Magnetic Interference
In a world where electronic devices and sensitive measurements are commonplace, magnetic shielding techniques emerge as protectors, channeling magnetic fields away from harm’s reach. These techniques safeguard electronics from interference and ensure accurate results in medical and scientific settings.
Predicting Magnetic Phenomena: Delving into Maxwell’s Equations and Numerical Simulations
Magnetic fields, seemingly invisible forces that permeate our surroundings, play a pivotal role in various technological and scientific applications. Understanding their behavior is crucial for harnessing their power. Enter Maxwell’s equations, the governing principles that describe the fundamental laws of electromagnetism. These equations provide a framework for predicting and analyzing magnetic field patterns.
Numerical techniques, such as finite element analysis, offer powerful tools for simulating magnetic fields in complex geometries. By discretizing the problem into smaller elements, these techniques solve the governing equations to obtain accurate solutions. This computational approach enables engineers and scientists to analyze magnetic fields in situations where analytical solutions are intractable.
With the aid of Maxwell’s equations and numerical simulations, we can delve into the intricate world of magnetic fields. These tools allow us to predict magnetic behavior, optimize designs, and gain a deeper understanding of electromagnetic phenomena.
Qualitative and Quantitative Analysis:
- Explain magnetic field mapping and its techniques.
- Quantify magnetic flux measurements.
- Highlight the role of magnetic resonance imaging (MRI) in medical imaging.
Unveiling the Secrets of Magnetic Fields: A Journey into Quantitative and Qualitative Analysis
In our exploration of the fascinating realm of magnetic fields, we delve into the realm of quantitative and qualitative analysis, where we unravel the mysteries of measuring and visualizing their presence. From intricate field mapping to precise flux measurements, we unlock the secrets of these invisible forces.
Mapping Magnetic Fields: A Window into the Invisible
Magnetic field mapping is the art of绘制the distribution of magnetic fields in physical space. Using various techniques, such as Hall probes and fluxgate magnetometers, we can visualize the intricate patterns created by magnets, current-carrying conductors, and other magnetic objects. This mapping process allows us to understand the shape, direction, and strength of magnetic fields, providing valuable insights into their behavior.
Quantifying Magnetic Flux: Measuring the Strength of Fields
Magnetic flux, a measure of the strength of a magnetic field, is akin to the flow of an invisible fluid. We employ specialized flux meters to precisely quantify this flow, capturing the amount of magnetic field passing through a given area. By understanding the flux distribution, we gain a deeper knowledge of the forces exerted by magnets and the magnetic properties of materials.
The Role of MRI: A Medical Breakthrough
Magnetic resonance imaging (MRI) stands as a transformative technology in medical imaging, harnessing the principles of magnetic fields and radio waves to probe the depths of the human body. By manipulating magnetic fields, we can reveal detailed anatomical structures, diagnose diseases, and monitor physiological processes. MRI plays a critical role in healthcare, providing invaluable insights into our health and well-being.
By mastering these techniques of quantitative and qualitative analysis, we gain a profound understanding of magnetic fields. We unravel their mysteries, harness their power, and apply them in countless technological and medical applications. From MRI scans to magnetic levitation, the exploration of magnetic fields continues to shape our world, revealing the hidden wonders that lie beyond our sight.
Simplifying Complex Magnetic Fields: A Guide to Understanding
Navigating the complexities of magnetic fields can be daunting, but with the right techniques, you can break down even the most challenging scenarios into manageable components. This guide will delve into three key approaches to simplifying complex magnetic fields: approximations, equivalent magnetic circuits, and lumped parameter models.
Magnetic Field Approximations
When dealing with complex magnetic fields, approximations can provide a useful starting point for analysis. These approximations simplify field calculations by assuming certain conditions or neglecting minor details. For instance, assuming uniform fields in a specific region can greatly reduce the computational burden. However, it’s crucial to recognize the limitations of approximations and their potential impact on accuracy.
Equivalent Magnetic Circuits
Another effective simplification technique involves creating equivalent magnetic circuits. These circuits model magnetic fields using electrical circuit elements, such as resistors and inductors. By replacing complex field distributions with equivalent circuits, engineers can leverage familiar electrical circuit analysis methods to obtain approximate magnetic field solutions. This approach is particularly useful for analyzing magnetic circuits with nonlinear materials.
Lumped Parameter Models
Finally, lumped parameter models offer a simplified representation of magnetic fields by averaging field values over a defined region. These models assign a single value to represent the entire field distribution within a given volume. Lumped parameter models are particularly useful for analyzing low-frequency magnetic fields, where the field variations are relatively small over short distances.
Understanding these simplification techniques empowers engineers and scientists to tackle complex magnetic field problems with confidence. By breaking down fields into manageable components, approximations, equivalent magnetic circuits, and lumped parameter models provide valuable tools for gaining insights into magnetic field behavior and finding practical solutions.
Interactive Simulations: A Gateway to Unraveling Magnetic Mysteries
Embark on a magnetic journey with interactive simulations that bring the complexities of magnetic fields to life. These tools provide a captivating way to visualize and interact with the enigmatic forces that shape our world.
Interactive Field Line Explorations:
Immerse yourself in the intricate dance of magnetic field lines. These simulations allow you to visualize the direction and strength of magnetic fields, painting a symphony of lines that guide charged particles like celestial dancers.
Visualizing Magnetic Forces:
Witness the power of the Lorentz force as charged particles pirouette through magnetic fields. Simulations let you adjust field strength and particle velocity, providing a hands-on exploration of the forces at play.
Magnetic Torque and Dipole Moments:
Discover the secrets of magnetic dipole moments and torque. Simulations bring to life the rotation of objects in magnetic fields, demonstrating how these forces interact and influence the behavior of matter.
Animations for Enhanced Understanding:
Complement your simulations with vibrant animations that bring abstract concepts to life. See how current-carrying wires generate magnetic fields, how magnets attract and repel, and how magnetic resonance imaging (MRI) paints a picture of the unseen.
Interactive Simulations: A Magnetic Playground for Curiosity:
Interactive simulations are not just educational tools; they are portals to a world of magnetic wonders. Engage with these simulations and let your curiosity soar as you delve into the enigmatic realm of magnetism.