“Unveiling Evolutionary Advancements: Beyond Darwin’s Natural Selection”
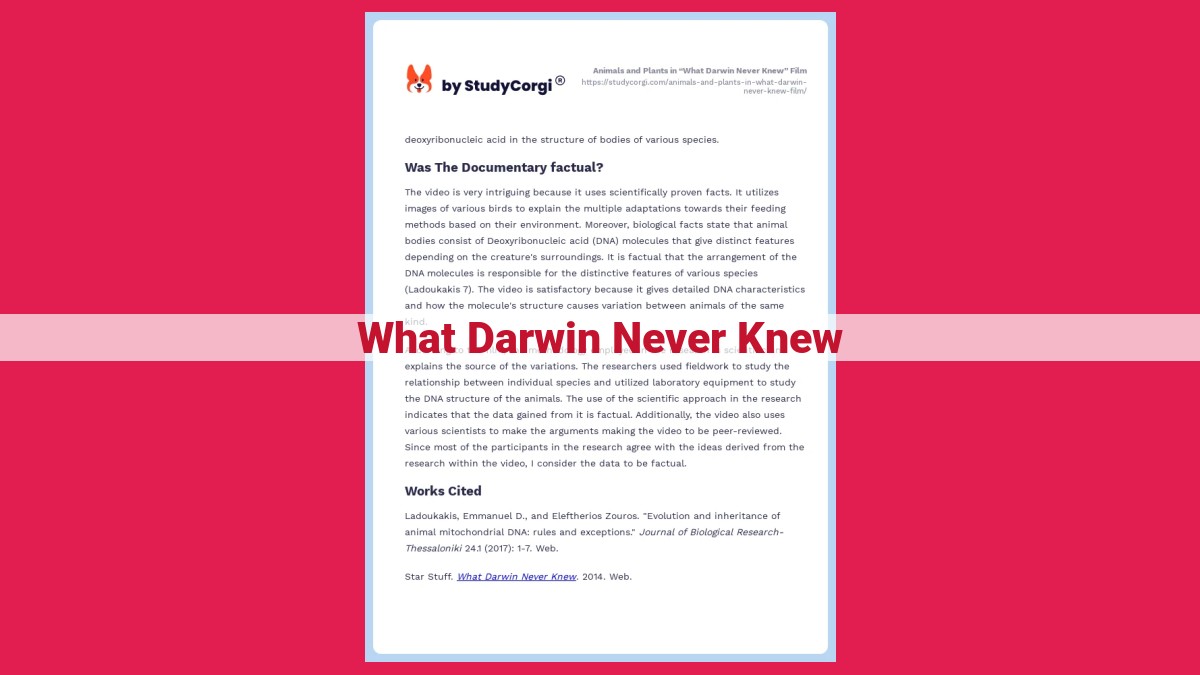
“What Darwin Never Knew” unveils the advancements in evolutionary science beyond Darwin’s original theory of natural selection. It explores the influence of genetic drift, gene flow, mutation, recombination, and developmental plasticity on genetic diversity and evolution. The book delves into epigenetic modifications, symbiosis, horizontal gene transfer, and coevolution, highlighting the interconnectedness of species and the dynamic nature of evolution.
Understanding Natural Selection: The Driving Force of Evolution
In the tapestry of life, evolution unfolds as a grand narrative of change and adaptation. At its core lies a powerful force called natural selection, the driving engine behind the remarkable diversity of species that grace our planet.
Imagine a vast and ancient forest. Amidst the lush undergrowth, a tiny population of beetles scuttles about. Each beetle possesses a unique set of traits, a genetic blueprint inherited from its parents. In this leafy realm, survival depends on finding food and avoiding predators.
Some beetles stumble upon a patch of succulent leaves, providing them with an ample food source. Their advantageous trait of keen eyesight grants them a competitive edge. As they feast, other beetles with less acute vision struggle to locate food, falling victim to predators.
Over time, the beetles with better eyesight outlive and outbreed their less gifted counterparts. Their heritable trait, passed down from generation to generation, becomes more prevalent in the population. This is the essence of natural selection: individuals with traits that enhance their survival and reproduction have a selective advantage.
As natural selection operates, populations gradually accumulate favorable traits that improve their adaptation to their environment. It is a relentless process, shaping and reshaping the genetic makeup of species over countless generations, giving rise to the astonishing variety of life that surrounds us.
Genetic Drift: The Dance of Chance and Diversity
In the vast tapestry of life, evolution weaves its intricate patterns through a delicate dance of natural forces. Genetic drift, a seemingly random yet powerful player in this symphony, holds the key to shaping the intricate threads of genetic diversity.
Imagine a small island, home to a population of finches with subtly different beak sizes. A sudden storm strikes, decimating a large portion of the population. By sheer luck, the surviving finches happen to possess the most advantageous beak size for the island’s dwindling food supply. Chance events, like this catastrophic storm, can dramatically alter the genetic makeup of a population.
The smaller the population, the more pronounced the effects of genetic drift become. As the number of individuals dwindles, the likelihood of losing valuable genetic variation increases. Conversely, in large populations, these random fluctuations are mitigated by the sheer abundance of genetic diversity. Over time, genetic drift can either fix advantageous traits or lead to the loss of genetic variation, potentially shaping the destiny of entire species.
Gene Flow: Connecting Genetic Pools
In the tapestry of evolution, gene flow plays a crucial role in shaping the genetic diversity of populations. It’s the movement of genetic material between populations, like a bridge that connects genetic islands, allowing for the exchange of beneficial traits and the enrichment of genetic variation.
Migration is a key driver of gene flow. When individuals move from one population to another, they carry with them a unique genetic imprint. This influx of new genetic material introduces traits that may be advantageous in the recipient population, promoting adaptation to local conditions.
Mating between individuals from different populations also contributes to gene flow. When genetic material from distinct lineages intertwines, it creates a diverse pool of genetic combinations. This increased genetic diversity provides a broader spectrum of potential traits, which can be vital for population survival and adaptability.
Gene flow has a profound impact on the evolution of species. It helps break down genetic barriers between populations, reducing the likelihood of genetic isolation and the emergence of subspecies. By fostering genetic exchange, gene flow ensures the continuous interplay of genetic material that fuels evolutionary change.
Mutation: Fueling Genetic Variation
Every species, including humans, is unique due to the genetic diversity within its population. Mutations play a crucial role in creating this diversity, serving as the driving force behind evolutionary change. They are random alterations in the DNA sequence that can be beneficial, harmful, or neutral to an organism’s survival.
Types of Mutations
There are several types of mutations, each with its potential impact on genetic variation:
- Single Nucleotide Polymorphism (SNP): A change in a single nucleotide, the building blocks of DNA. SNPs are the most common type of mutation and can affect gene expression or regulation.
- Insertion or Deletion: The addition or removal of one or more nucleotides, which can alter the reading frame and disrupt gene function.
- Copy Number Variation (CNV): Changes in the number of copies of a particular gene or region, which can lead to increased or decreased gene expression.
- Chromosomal Mutations: Larger-scale changes involving the structure or number of whole chromosomes. These can have significant consequences, such as Down syndrome or Turner syndrome.
How Mutations Create Genetic Diversity
Mutations introduce new genetic variants into a population, increasing the diversity of genes and traits within the species. These variants can then be passed on to offspring, contributing to the genetic makeup of future generations.
Beneficial mutations can enhance an individual’s survival, such as conferring resistance to disease or improved adaptation to environmental changes. Harmful mutations, on the other hand, can lead to genetic disorders or reduce an individual’s fitness. While most mutations have little impact on survival, the cumulative effect of mutations over time can drive significant evolutionary change.
Mutations are essential for the evolution of life, providing the raw material for natural selection to work upon. By introducing new genetic variants, mutations fuel genetic diversity and set the stage for ongoing adaptation and the formation of new species. Without mutation, evolution would be impossible, and life on Earth would remain stagnant.
Recombination: Shuffling the Genetic Deck
In the tapestry of life, the dance of genetic recombination weaves a vibrant masterpiece that fuels the symphony of evolution. This intricate process unfolds within the enigmatic realm of meiosis, a cellular ballet that orchestrates the creation of gametes—the sperm and eggs that carry life’s genetic legacy forward.
Meiosis: A Tale of Cellular Transformation
Meiosis, an evolutionary virtuoso, transforms diploid cells—cells with two sets of chromosomes—into haploid cells, each containing only half the genetic material. This delicate dance unfolds in two distinct phases, meiosis I and meiosis II.
Meiosis I: A Chromosomal Tango
As meiosis I commences, homologous chromosomes, identical twins in the genetic realm, pair up and undergo a graceful dance called crossing-over. This genetic waltz allows sections of DNA to exchange places, shuffling the genetic deck and creating new combinations. These reconfigured chromosomes then separate, each carrying a unique blend of genetic information.
Meiosis II: The Final Split
Meiosis II completes the separation process. The haploid cells, now with their single set of chromosomes, undergo a final round of division. The result: four unique gametes, each harboring a distinctive genetic blueprint that embodies the legacy of countless ancestors.
Evolution’s Genetic Jigsaw
Recombination is the engine that drives genetic diversity, the foundation upon which natural selection weaves its evolutionary tapestry. Through the kaleidoscope of genetic recombination, novel traits arise, providing the raw material for adaptation and survival in the ever-changing dance of life.
Explain how the environment can influence the expression of genes and the development of different phenotypes.
Developmental Plasticity: Phenotypes Shaped by Environment
The intricate interplay between genetics and the environment is vividly illustrated in the concept of developmental plasticity. Like a sculptor shaping a piece of clay, the environment influences the expression of genes, molding the development of different phenotypes within a single genotype.
When conditions change, the environment can trigger epigenetic modifications, altering the accessibility of genes without altering their underlying DNA sequence. These modifications may activate dormant genes or silence others, shaping the development of new cellular pathways.
For instance, imagine a species of butterflies whose larvae respond to food availability. In times of plenty, larvae develop into large, brightly colored adults with wide wingspans, benefiting from increased mating success and predator deterrence. However, during food scarcity, the same larvae morph into smaller, dull-colored adults with narrower wings, ensuring survival despite limited resources.
Developmental plasticity enables organisms to adapt to diverse environments without the need for immediate genetic changes. Like a chameleon changing its hue, it provides the flexibility to adjust phenotypes in response to changing conditions, optimizing survival and maximizing reproductive success.
Epigenetics: Unlocking the Hidden Code of Gene Expression
Unveiling the intricate tapestry of life, our understanding of evolution extends far beyond the realm of basic genetics. Epigenetics emerges as a captivating chapter, where the environment whispers its secrets to our DNA, shaping our traits and influencing our destiny.
Like an invisible conductor, epigenetics orchestrates the symphony of gene expression without altering the underlying genetic code. Through remarkable mechanisms, it flips the switches of our genes, determining which will play and which will remain silent. These modifications, etched into the epigenetic landscape like ancient runes, can be passed down through generations, leaving an enduring legacy.
One key epigenetic mechanism involves DNA methylation, where chemical groups attach to DNA’s “C” nucleotides, silencing gene expression. Conversely, histone modification alters the protein reels around which DNA coils, either loosening or tightening its structure to promote or inhibit gene accessibility.
These epigenetic marks dance in response to our environment, like notes on a sheet of music. Environmental triggers, such as nutrition, stress, and exposure to toxins, can leave their imprint on our epigenetic landscape. The consequences are profound: they can affect our behavior, susceptibility to diseases, and even our longevity.
Epigenetic modifications provide a crucial layer of adaptability to our genome. They allow us to adapt to changing conditions without altering our genetic blueprint. This flexibility is essential for survival, as it enables us to make quick adjustments to the ebb and flow of our surroundings.
In conclusion, epigenetics is a fascinating and dynamic field of study, unveiling the hidden code that influences our lives. By understanding the mechanisms involved in epigenetic modifications, we gain insights into the interplay between our genes and our environment, unlocking the potential for personalized medicine and a deeper comprehension of the complexities of life itself.
Symbiosis: Interconnected Species
In the grand tapestry of life, organisms rarely exist in isolation. They form intricate relationships with one another, ranging from fleeting encounters to lifelong partnerships. These symbiotic interactions have profoundly shaped the evolutionary journey of countless species.
Mutualism: A Harmonious Dance
Mutualism is a beautiful dance where both participants benefit. Consider the symbiotic relationship between nitrogen-fixing bacteria and legumes. These bacteria reside within the roots of legumes, extracting nitrogen from the air and converting it into a form that the plant can utilize. In return, the plant provides the bacteria with shelter and nutrients. This partnership enhances the soil fertility, benefiting not only the legumes but also other surrounding plants.
Commensalism: A One-sided Benefit
In commensalism, one species reaps the benefits without harming the other. A prime example is the relationship between sea anemones and clownfish. The clownfish find refuge within the anemones’ venomous tentacles, protected from predators. The anemones, on the other hand, remain largely unaffected by this association.
Parasitism: A One-sided Exploitation
Unlike mutualism and commensalism, parasitism is a one-sided affair. Parasites derive nourishment or other resources from their hosts, often at the expense of the host’s health. Ticks, for instance, attach themselves to animals and feed on their blood, potentially transmitting diseases. The host, while suffering from the parasite’s presence, does not directly benefit from the relationship.
Symbiosis: A Catalyst for Evolutionary Change
Symbiotic interactions can drive significant evolutionary changes. Over time, organisms may adapt to exploit these relationships more efficiently. In mutualistic relationships, for example, the partners may evolve specialized structures or behavioral traits that enhance their cooperation. Parasites, on the other hand, may develop strategies to overcome their hosts’ defenses, leading to an evolutionary arms race.
Symbiotic relationships are a testament to the interconnectedness of life. From the harmonious dance of mutualism to the parasitic exploitation of hosts, these interactions have played a crucial role in shaping the diversity and complexity of the natural world. By fostering interdependence, symbiosis allows species to adapt, survive, and thrive in a constantly changing environment.
Horizontal Gene Transfer: Sharing the Code of Life
In the grand tapestry of evolution, genetic material isn’t always passed down vertically from parents to offspring. Sometimes, genes take a more unconventional route, traveling horizontally between organisms of different species. This remarkable phenomenon, known as horizontal gene transfer (HGT), has the power to shake up the genetic landscape and drive evolutionary change.
Unlike traditional inheritance, HGT allows organisms to acquire new genes from their environment, bypassing the usual reproductive processes. This genetic exchange occurs through various mechanisms, each with its unique implications. One common pathway is through viruses. These infectious agents can carry genes from one host to another, introducing new genetic material into the recipient’s genome. This mechanism has played a pivotal role in the spread of antibiotic resistance among bacteria, as viruses can ferry these resistance genes between different bacterial populations.
Bacterial Transformation: Swapping Genes in a Fluid Environment
Another mechanism of HGT is bacterial transformation, where bacteria take up DNA from their surrounding environment. This process is particularly common in bacteria that live in close-knit communities, such as those found in soil or aquatic habitats. When a bacterium dies and releases its DNA into the environment, neighboring bacteria may absorb this genetic material and incorporate it into their own genomes. Through transformation, bacteria can acquire new genes that enhance their survival, such as antibiotic resistance or the ability to metabolize unusual compounds.
Conjugation: A Direct Genetic Link
Conjugation is a more intimate form of HGT, occurring when two bacteria physically connect through a thin, tube-like structure called a pilus. This pilus acts as a conduit for the transfer of genetic material from the donor bacterium to the recipient bacterium. Plasmids, circular pieces of DNA that carry genes not found on the main bacterial chromosome, are commonly exchanged through conjugation. Plasmids often confer beneficial traits, such as antibiotic resistance or the ability to degrade toxic compounds.
HGT has profound implications for evolution. It allows organisms to acquire new genes that can enhance their fitness in response to environmental challenges. By sharing genetic material, organisms can adapt more rapidly to changing conditions and explore new ecological niches. This process has played a significant role in the evolution of antibiotic resistance, the emergence of new pathogens, and the development of symbiotic relationships between different species.
Coevolution: Dance of Adaptation
In the intricate tapestry of life, species are not isolated entities but rather interconnected participants in a grand evolutionary saga. The interactions between species can spark a reciprocal dance of adaptation, driving extraordinary evolutionary change.
Imagine two species locked in an evolutionary arms race: the predator and its prey. As the predator hones its hunting skills, the prey develops keen senses and defensive mechanisms to evade capture. This interplay of predation and avoidance fuels an escalating cycle of adaptation.
Another example of coevolution is the remarkable relationship between flowering plants and their pollinators. Over time, flowers have evolved flamboyant blooms and sweet nectar to attract pollinators, while pollinators have developed specialized mouthparts and sensory organs to efficiently gather nectar. This mutually beneficial partnership ensures the survival and reproduction of both species.
Coevolution can also extend beyond predator-prey and symbiotic relationships. For instance, the presence of a certain plant species may influence the abundance and behavior of herbivores, which in turn affects the population dynamics of competing plant species. This complex web of interactions can shape the structure and composition of entire ecosystems.
Coevolution is a testament to the interconnectedness of life and the relentless drive of evolution to adapt and thrive. It is a process that has shaped the diversity and complexity of life on Earth, from the simplest microorganisms to the most magnificent creatures. By understanding the dynamics of coevolution, we gain a deeper appreciation for the intricate and beautiful tapestry of life.