Understanding Systematic Nomenclature: Unraveling The Name “Lead(Ii) Oxide”
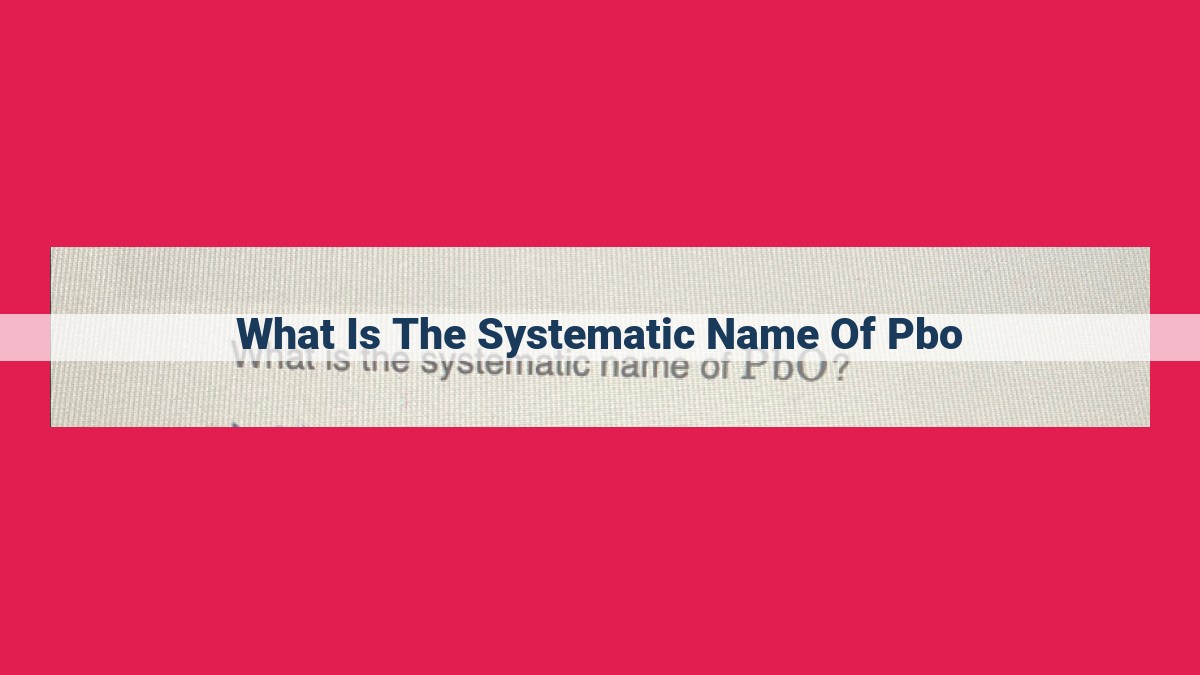
The systematic name of PbO is lead(II) oxide. In chemical nomenclature, the systematic name of a compound indicates the elements present and their oxidation states. PbO contains lead (Pb) and oxygen (O), and the Roman numeral (II) specifies that lead is in the +2 oxidation state. The name “lead(II) oxide” clearly identifies the compound as a binary oxide of lead with a specific oxidation state. This systematic naming convention ensures uniformity and facilitates clear communication among chemists and other scientists.
Chemical Nomenclature: The Language of Chemistry
Imagine trying to communicate with someone without a shared language. It would be nearly impossible to convey complex ideas! In the world of science, chemical nomenclature serves as the common language that allows scientists around the globe to describe and identify chemical substances with precision.
What is Chemical Nomenclature?
Chemical nomenclature is a system of rules and guidelines that govern the naming of chemical compounds. It provides a standardized method for assigning unique and meaningful names to substances, making it easier for scientists to discuss, research, and apply them.
Types of Chemical Nomenclature
There are several different types of chemical nomenclature, each with its own advantages and disadvantages. The International Union of Pure and Applied Chemistry (IUPAC) has established a set of rules for systematic nomenclature, which is the most widely used today. Traditional nomenclature uses common names for certain compounds, while trivial nomenclature employs non-systematic names that have historical or practical significance.
Importance of Standardized Naming
A standardized naming system is crucial for scientists to effectively communicate about chemical substances. It ensures that everyone is referring to the same compound, regardless of their location or language. This facilitates accurate and efficient research, collaboration, and the sharing of scientific knowledge. Moreover, a well-defined nomenclature system promotes clarity and consistency in scientific literature, making it easier for readers to understand and interpret chemical information.
Oxidation States: Unlocking the Secrets of Redox Reactions
In the realm of chemistry, understanding oxidation states holds the key to comprehending redox reactions, a fascinating interplay between chemical substances that transforms their identity. Oxidation state assigns a numerical value to an atom within a compound, representing the hypothetical charge it would have if all bonds were ionic. This concept serves as a crucial tool for chemists, unraveling the mysteries of chemical interactions.
Determining oxidation states is a fundamental skill in chemistry. To embark on this journey, we delve into the oxidation number method, where the sum of oxidation states for all atoms in a neutral compound must equal zero, and the sum of oxidation states for all atoms in a polyatomic ion must equal its overall charge. These rules guide us in calculating oxidation states, empowering us to comprehend the intricate world of chemical bonding.
The significance of oxidation states extends beyond merely assigning numerical values. They serve as powerful predictors of an atom’s behavior in redox reactions, where oxidation involves the loss of electrons, while reduction involves the gain of electrons. By examining oxidation states before and after a reaction, we can deduce which atoms undergo oxidation or reduction, unlocking insights into the flow of electrons and the chemical transformations that occur.
Redox reactions are not mere abstract concepts; they play a pivotal role in countless natural and industrial processes. From the combustion of fuels to the intricate reactions within living organisms, redox reactions orchestrate a symphony of chemical transformations. Understanding oxidation states empowers us to delve deeper into these processes, unraveling their mysteries and harnessing their potential for technological advancements.
Binary Compounds: The Basics
Binary compounds are chemical substances that contain only two different elements. These elements can be metals, nonmetals, or a combination of both. Binary compounds are ubiquitous in our world, from the salt we sprinkle on our food to the water we drink.
Classification of Binary Compounds
Binary compounds are classified into two main types: ionic and molecular.
Ionic binary compounds are formed when a metal reacts with a nonmetal. The metal loses one or more electrons to the nonmetal, creating positively charged ions (cations) and negatively charged ions (anions). These ions are then attracted to each other by electrostatic forces, forming an ionic bond. Ionic binary compounds are typically hard, brittle, and have high melting and boiling points. They also dissolve easily in water to form electrically conducting solutions.
Molecular binary compounds are formed when two nonmetals react with each other. They are composed of molecules, which are neutral entities held together by covalent bonds. Covalent bonds are formed when atoms share electrons. Molecular binary compounds are typically gases, liquids, or low-melting solids. They are generally soft, have low melting and boiling points, and do not dissolve in water.
Properties and Examples of Ionic and Molecular Binary Compounds
Ionic binary compounds typically have the following properties:
- High melting and boiling points: The strong electrostatic forces between the ions require a lot of energy to break.
- Hard and brittle: The ions are tightly packed together, making the compound difficult to deform.
- Electrical conductivity: Ionic binary compounds dissolve in water to form electrically conducting solutions. The ions are free to move in solution, carrying electrical charges.
Examples of ionic binary compounds include sodium chloride (NaCl), potassium iodide (KI), and calcium oxide (CaO).
Molecular binary compounds typically have the following properties:
- Low melting and boiling points: The covalent bonds between the atoms are weaker than the electrostatic forces in ionic compounds, so less energy is required to break them.
- Soft and volatile: Molecular binary compounds are easily deformed and evaporate readily.
- Poor electrical conductivity: Molecular binary compounds do not dissolve in water to form electrically conducting solutions. The molecules are neutral and do not carry electrical charges.
Examples of molecular binary compounds include hydrogen chloride (HCl), carbon dioxide (CO2), and water (H2O).
Lead: A Complex Metal with Both Benefits and Risks
Properties and Applications of Lead
Lead is a heavy metal known for its silvery-white appearance and malleable nature. Its high density and low melting point make it an ideal material for various industrial applications:
- Storage batteries: Lead-acid batteries are commonly used in vehicles and energy storage systems due to their reliability and cost-effectiveness.
- Radiation shielding: Lead’s ability to absorb radiation effectively makes it crucial for use in medical, nuclear, and industrial settings.
- Construction: Lead is incorporated into roofing, sealants, and pipes due to its resistance to corrosion and durability.
Toxicity and Environmental Concerns Associated with Lead
While lead offers valuable properties, it also poses significant environmental and health risks:
- Neurotoxicity: Lead exposure, primarily through contaminated air, food, or soil, can severely harm the nervous system, especially in children.
- Developmental problems: Even low levels of lead exposure can impair cognitive development, behavior, and academic performance.
- Environmental contamination: Lead mining and manufacturing processes release harmful lead emissions into the air and water, which can accumulate in ecosystems and pose a threat to wildlife and human health.
Mitigation Strategies for Lead Pollution
Recognizing the dangers of lead exposure, governments and organizations are implementing measures to reduce its impact:
- Stricter regulations: Governments have established stringent limits for lead content in consumer products, fuels, and industrial emissions to minimize environmental contamination.
- Lead abatement: Old buildings, which often contain lead paint, are undergoing removal and replacement programs to prevent lead poisoning from chipping and dust.
- Public awareness: Educational campaigns and community outreach efforts are raising awareness about the risks of lead exposure and promoting preventive measures.
Lead is a versatile metal with a range of applications, but its toxicity and environmental impact demand careful consideration. By implementing mitigation strategies and increasing public awareness, we can harness the benefits of lead while minimizing its potential hazards, ensuring a healthier future for both humans and the environment.
Oxygen: The Essence of Life and Beyond
Significance of Oxygen for Life on Earth
Oxygen is the breath of life. It is essential for the survival of almost all aerobic organisms, including humans, animals, and plants. Oxygen plays a pivotal role in cellular respiration, the process by which cells convert glucose into energy. This energy is the fuel that powers our bodies and allows us to function. Without oxygen, life as we know it would not be possible.
Role of Oxygen in Respiration, Photosynthesis, and Combustion
Oxygen is not just essential for life; it also plays a crucial role in many natural processes. It is the final electron acceptor in the electron transport chain during respiration, enabling the efficient production of ATP, the energy currency of cells.
Moreover, oxygen is indispensable for photosynthesis, the process by which plants convert sunlight into food. Plants release this food as oxygen, which forms the basis of the entire food chain.
Additionally, oxygen is essential for combustion, the process that fuels everything from fires to power plants. Without oxygen, combustion would not occur, and we would not have access to many of the conveniences we rely on today.
Environmental Implications of Changes in Oxygen Levels
Changes in oxygen levels in the environment can have profound implications. Decreases in oxygen levels, such as those caused by pollution or climate change, can lead to hypoxia, a condition of oxygen deprivation that can have detrimental effects on aquatic life and ecosystems.
Conversely, increased oxygen levels can lead to hyperoxia, which can also be harmful to living organisms. Therefore, maintaining optimal oxygen levels is crucial for the health of our planet.
In conclusion, oxygen is an indispensable element that sustains life on Earth and fuels countless natural processes. Its importance cannot be overstated, and we must strive to protect and preserve its delicate balance for generations to come.