Understanding The Freezing Point Of Oxygen: A Guide To Its Properties, Applications, And Implications
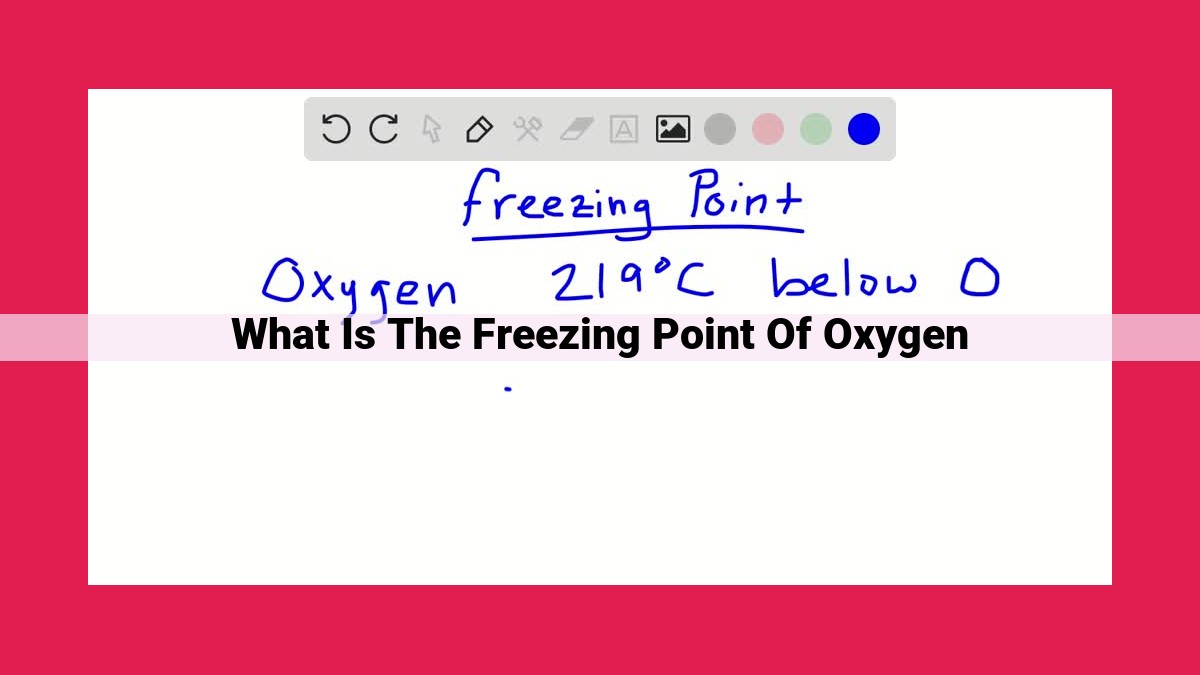
The freezing point of oxygen, the temperature at which it solidifies, is a fundamental property influenced by pressure and impurities. The phase diagram of oxygen reveals the coexistence of solid, liquid, and gas phases at different temperatures and pressures. The triple point, where all three phases are in equilibrium, determines the freezing point at a specific pressure. Impurities and surface effects can alter the freezing point, impacting applications such as cryopreservation and food preservation. Understanding the freezing point of oxygen is crucial for manipulating its properties and utilizing it in various scientific and industrial processes.
- Introduce the topic of the freezing point of oxygen and its significance.
- Provide a brief overview of the physical and chemical properties of oxygen.
The Enigmatic Freezing Point of Oxygen: Exploring the Secrets of the Life-Sustaining Element
Oxygen, the very essence of life, holds within its molecular structure a captivating secret: its freezing point. This extraordinary phenomenon plays a vital role in shaping our world, from the preservation of biological materials to the extension of food shelf life.
An Essential Element with Unique Qualities
Oxygen, a diatomic gas, is characterized by its distinctive colorless, odorless, and tasteless nature. It comprises approximately 21% of the Earth’s atmosphere, making it the third most abundant element. Its presence is crucial for sustaining life and supporting numerous industrial processes.
In its solid form, oxygen appears as a pale blue crystalline substance. This unique freezing point is attributed to the element’s covalent bonds and intermolecular forces. Understanding the factors that govern oxygen’s freezing point unveils the intricate workings of this essential element.
Understanding Freezing Point: A Phase Transition Phenomenon
When liquids freeze, they undergo a magical transformation from flowing streams to solid states. This transition, known as freezing point, marks the temperature at which the liquid’s molecules lose their freedom of movement and become locked in a crystalline structure.
Phase transitions are fascinating processes that occur when substances change from one state to another. These transitions are influenced by various factors, primarily temperature. As a liquid cools, its molecules slow down. At the freezing point, the molecules’ kinetic energy drops to the point where they can no longer overcome the attractive forces between them, causing them to form a solid structure.
This phenomenon is not limited to water; every substance has its own unique freezing point. Oxygen, the vital gas we breathe, is no exception. Understanding the freezing point of oxygen is essential not only for scientific exploration but also for practical applications in fields such as cryopreservation and food preservation.
Delving into the Phase Diagram of Oxygen: Unraveling the Temperature-Pressure Relationships
- Introduce the phase diagram of oxygen and its importance in understanding its phase transitions.
- Discuss the different phases of oxygen (solid, liquid, and gas) and the conditions under which they exist.
Delving into the Phase Diagram of Oxygen: Unraveling the Temperature-Pressure Dance
Imagine a magical chart called a phase diagram, where the secrets of oxygen’s transformations unfold. This diagram dances with two variables: temperature and pressure.
The Three Faces of Oxygen
Within this phase diagram’s landscape, three distinct characters emerge: solid, liquid, and gas oxygen. Each phase has its own realm, separated by boundaries that shift and sway depending on temperature and pressure.
Solid Oxygen: A Brittle Haven
At extremely low temperatures and high pressures, oxygen takes on a solid form, a crystalline haven where molecules pack closely together. This realm is a world of rigidity, where oxygen’s dance is constrained.
Liquid Oxygen: A Flowing Interlude
As temperature rises while pressure remains high, oxygen transforms into a liquid. In this liquid realm, molecules gain mobility, flowing and swirling freely within their confined space.
Gaseous Oxygen: Breaking Free
When both temperature and pressure are low, oxygen reigns supreme in its gaseous form. Here, molecules dance unbound, soaring through space with newfound freedom.
The Triple Point: A Place of Perfect Balance
However, there exists a magical point where all three phases coexist harmoniously, each playing its part in the dance of oxygen’s transformation. This point, known as the triple point, is a place of perfect balance, where temperature, pressure, and the presence of all three phases create a delicate equilibrium.
Unlocking the Secrets of Oxygen’s Freezing Point: Delving into the Triple Point
In the realm of matter’s transformations, the freezing point reigns supreme as the pivotal moment when a liquid surrenders its fluidity and embraces solidity. For oxygen, this transition holds profound significance not only for its scientific allure but also for its practical applications. One key to unraveling the mysteries of oxygen’s freezing point lies in understanding the triple point.
The triple point is the extraordinary point where three phases of a substance – solid, liquid, and gas – coexist in equilibrium. For oxygen, this equilibrium point occurs at a specific temperature of -218.4 degrees Celsius (-361.1 degrees Fahrenheit) and a pressure of 0.0015 atmospheres.
At the triple point, oxygen’s molecules engage in a delicate dance, delicately balancing their desire to maintain their liquid state with the pull towards becoming a solid. This equilibrium is crucial because it determines the precise freezing point of oxygen. Below the triple point temperature, oxygen transforms into a solid, while above it, it remains a liquid.
The significance of the triple point extends beyond mere coexistence. It serves as a cornerstone for understanding other thermodynamic properties of oxygen, such as its heat of fusion and vapor pressure. These properties are essential in diverse fields, ranging from cryopreservation to industrial gas applications.
In the world of scientific exploration, the triple point has proven to be an invaluable tool. It enables researchers to determine the freezing point of oxygen with remarkable accuracy, paving the way for advanced investigations into its behavior and properties.
Moreover, the triple point finds practical relevance in industries such as cryogenics, where the preservation of biological materials at ultra-low temperatures relies on precise control of oxygen’s phase transitions. By manipulating pressure and temperature around the triple point, scientists can optimize cryopreservation techniques, ensuring the long-term viability of cells and tissues.
In essence, the triple point of oxygen represents a gateway into understanding the substance’s thermodynamic behavior. It serves as a critical reference point for researchers and practitioners alike, offering insights into the remarkable properties of this life-sustaining gas. By embracing the equilibrium dance of the triple point, we unlock the secrets of oxygen’s freezing point and delve into a world of scientific wonders.
Pressure Matters: Exploring the Intriguing Relationship between Pressure and Freezing Point
Imagine you have a glass of water. If you put it in the freezer, it will eventually turn into ice at 0 degrees Celsius (32 degrees Fahrenheit). But what if you change the pressure?
The Curious Case of Pressure and Freezing Point
It turns out that pressure has a profound effect on the freezing point of a substance. Generally speaking, as pressure increases, the freezing point also increases. This means that water will freeze at a higher temperature if you apply more pressure to it.
This phenomenon is not limited to water. It applies to oxygen as well. Oxygen’s freezing point at atmospheric pressure is -183 degrees Celsius (-301 degrees Fahrenheit). However, if you increase the pressure, the freezing point will rise.
Real-World Applications
This pressure-freezing point relationship has practical applications in various fields:
-
Cryopreservation: Preserving biological samples at ultra-low temperatures requires precise control of freezing conditions. Oxygen’s freezing point can be adjusted by manipulating pressure to ensure optimal preservation.
-
Food Preservation: Freezing techniques, such as flash freezing and freeze-drying, utilize the effect of pressure on freezing point to extend the shelf life of food products by inhibiting microbial growth.
Examples
-
Oxygen cylinders: Oxygen used in medical or industrial settings is stored in pressurized cylinders. The increased pressure helps maintain the oxygen in a liquid state, preventing it from freezing and blocking the flow.
-
Underwater diving: Divers use pressurized oxygen mixtures in their tanks to prevent oxygen from freezing at the higher pressures encountered underwater.
-
Ice skating: The weight of the skater increases the pressure on the ice, which lowers its freezing point and makes it easier to glide.
The relationship between pressure and freezing point is a captivating aspect of oxygen’s behavior. Understanding this phenomenon not only enriches our knowledge of thermodynamics but also has practical implications in fields such as cryopreservation, food preservation, and diving.
The Influence of Impurities and Surface Effects on Oxygen’s Freezing Point
As we delve deeper into the intricacies of oxygen’s freezing point, we encounter two intriguing factors that can significantly affect this behavior: impurities and surface effects.
Impurities: Imagine oxygen as a pristine lake, its surface undisturbed. When impurities, such as dust particles or dissolved gases, enter the water, they create imperfections that disrupt the lake’s smooth surface. Similarly, impurities dissolved in oxygen can disrupt the arrangement of its molecules, making it more difficult for them to form the crystalline structure that characterizes the solid phase. This depresses the freezing point, meaning that oxygen contaminated with impurities will freeze at a lower temperature than pure oxygen.
Surface effects: At the heart of freezing lies a nucleation process, where tiny crystals of the solid phase start to form. The surface of a container or the presence of foreign particles can provide preferential sites for nucleation, influencing the freezing point. Smooth, clean surfaces tend to promote nucleation and thus lower the freezing point, while rough or contaminated surfaces can hinder nucleation and elevate the freezing point.
These impurities and surface effects not only alter the temperature at which oxygen freezes but can also impact the quality and properties of the resulting solid. Understanding these factors is crucial in various applications, from cryopreservation to food preservation.
Experimental Techniques: Unveiling the Secrets of Freezing Point Determination
- Introduction to various experimental methods used to determine the freezing point of oxygen, such as thermal analysis, microscopy, and differential scanning calorimetry.
- Discuss the principles and applications of each technique.
Unveiling the Secrets of Oxygen’s Freezing Point: Experimental Techniques
The freezing point of oxygen, where the liquid transforms into a solid, holds great significance in various scientific and industrial applications. To unravel its intricacies, scientists employ an array of experimental techniques that provide invaluable insights into this phase transition phenomenon.
Thermal Analysis: Delving into Heat Flow
- Thermal analysis techniques, like differential scanning calorimetry, measure the heat flow associated with the freezing process. As oxygen solidifies, it releases heat energy that is detected by the instrument. This heat flow data enables researchers to pinpoint the exact temperature at which freezing occurs, revealing the precise freezing point.
Microscopy: Visualizing the Phase Change
- Microscopy techniques, such as cryo-electron microscopy, allow scientists to directly visualize the oxygen sample undergoing the freezing process. By observing the formation and growth of ice crystals in real-time, researchers can gather crucial information about the nucleation and crystallization behavior of oxygen.
Differential Scanning Calorimetry: Uncovering Energy Profiles
- Differential scanning calorimetry, a powerful thermal analysis technique, measures the difference in heat flow between a sample and a reference material. As oxygen freezes, it exhibits a characteristic endothermic peak on the DSC thermogram, indicating the absorption of heat energy during the transition. This peak corresponds to the freezing point and provides insights into the energetics of the process.
These experimental techniques, each offering unique perspectives, collectively empower scientists to decipher the intricate details of oxygen’s freezing point. From understanding the fundamental phase transition behavior to optimizing cryopreservation and food preservation techniques, these methods play a pivotal role in harnessing the power of oxygen for diverse scientific endeavors.
Cryopreservation: Unlocking the Secrets of Preserving Life with Oxygen’s Freezing Point
In the realm of scientific exploration, the freezing point of oxygen holds a remarkable significance, paving the way for transformative advancements in cryopreservation. This intricate process involves meticulously preserving biological materials, including cells, tissues, and even entire organisms, at ultra-low temperatures. By harnessing the unique properties of oxygen, scientists have unlocked the secrets to extending the viability of life beyond its natural boundaries.
Oxygen’s freezing point, a critical parameter in cryopreservation, plays a pivotal role in determining the success or failure of preserving biological samples. As liquid oxygen transitions into its solid state, it forms crystals that can potentially damage delicate cellular structures. However, by carefully controlling the freezing rate and temperature, scientists can minimize ice crystal formation, preserving the integrity of the biological material.
The freezing point of oxygen is also influenced by the presence of impurities and surface effects. Minute amounts of impurities can lower the freezing point, while surface effects can alter the nucleation process, further affecting the crystallization behavior. Understanding these factors is crucial for optimizing cryopreservation techniques and ensuring the highest viability of preserved samples.
Through rigorous experimentation, scientists have developed precise methods to determine the freezing point of oxygen. These techniques, ranging from thermal analysis to microscopy and differential scanning calorimetry, provide in-depth insights into the freezing behavior of oxygen and its impact on biological materials.
In the field of medicine, cryopreservation empowers researchers to preserve cells and tissues for extended periods. This capability has revolutionized the storage of human organs for transplant, fertility preservation, and the development of cell-based therapies. By harnessing the freezing point of oxygen, scientists are pushing the boundaries of medical advancements and improving the quality of life for countless individuals.
Cryopreservation also plays a vital role in food preservation. Freezing inhibits microbial growth and extends the shelf life of food products. By optimizing the freezing process and utilizing the freezing point of oxygen, the food industry can reduce waste and ensure the safety and quality of our food supply.
Exploring the thermodynamic properties of oxygen beyond its freezing point expands our understanding of this essential element. The ideal gas law governs the behavior of oxygen gas, and its vapor pressure and heat of fusion are critical in applications ranging from cryogenic refrigeration to aerospace engineering.
Delving into the intriguing world of oxygen’s freezing point unveils its profound impact on scientific research, medical advancements, and industrial processes. By harnessing the power of this remarkable phenomenon, we continue to push the boundaries of human ingenuity and transform the future of medicine, food preservation, and countless other fields.
Food Preservation: Extending Shelf Life with Oxygen’s Freezing Point
The freezing point of oxygen plays a pivotal role in preserving the freshness and extending the shelf life of food products. By harnessing the power of this fundamental property, we can effectively inhibit microbial growth and maintain the quality of our food supply.
Freezing food at temperatures below its freezing point immobilizes water molecules, preventing microbial activity responsible for spoilage. This process slows down enzymatic reactions and prevents the proliferation of bacteria and other microorganisms. As a result, frozen foods retain their nutritional value and freshness for longer periods.
Freeze-drying, another food preservation technique, relies on the sublimation of water from frozen food under vacuum conditions. By lowering the temperature below the freezing point of oxygen, we create an environment where water molecules directly transition from solid to gas without passing through the liquid phase. This technique minimizes cell damage and preserves the original texture and flavor of the food.
The freezing point of oxygen is not constant but can be influenced by factors such as pressure and the presence of impurities. Understanding these factors allows us to optimize freezing and freeze-drying conditions for different food items. By carefully controlling the temperature and pressure during food processing, we can effectively extend the shelf life of our food while maintaining its quality and nutritional value.
In conclusion, the freezing point of oxygen is a crucial parameter in food preservation. By leveraging this property, we can inhibit microbial growth, prevent spoilage, and extend the shelf life of our food products. This knowledge empowers us to enjoy safe and nutritious food for longer periods, reducing waste and promoting a sustainable food system.
Beyond Freezing Point: Delving into the World of Oxygen’s Thermodynamic Properties
- Introduce the ideal gas law and its relevance to understanding the behavior of oxygen gas.
- Explore other thermodynamic properties of oxygen, such as vapor pressure and heat of fusion, and their significance in various applications.
Beyond Freezing Point: Unveiling the Multifaceted Thermodynamic Properties of Oxygen
In our exploration of oxygen’s intriguing properties, we ventured beyond its freezing point to unravel the captivating world of thermodynamics. The ideal gas law, like an orchestra conductor, harmoniously governs the behavior of oxygen gas. This law elegantly describes the interrelationship between pressure, volume, temperature, and number of molecules in a gas.
Venturing further, we encounter the vapor pressure of oxygen, a measure of its tendency to vaporize. This property plays a crucial role in applications such as cryogenics, where ultra-low temperatures are employed to preserve biological materials. The higher the vapor pressure, the more readily oxygen transitions from its liquid to its gaseous state.
Another intriguing thermodynamic property is the heat of fusion, which quantifies the energy required to transform oxygen from a solid to a liquid at its melting point. This property governs the efficiency of freeze-drying techniques used to extend the shelf life of food products.
Oxygen’s thermodynamic properties extend far beyond its freezing point, revealing a tapestry of intricate relationships that shape its behavior in countless applications. These properties underpin everything from the preservation of life to the delicious preservation of our favorite foods. As we continue to unravel the secrets of oxygen, we uncover the profound influence it exerts on our world.