Understanding The Negative Charge Of The Hydroxide Ion (-1)
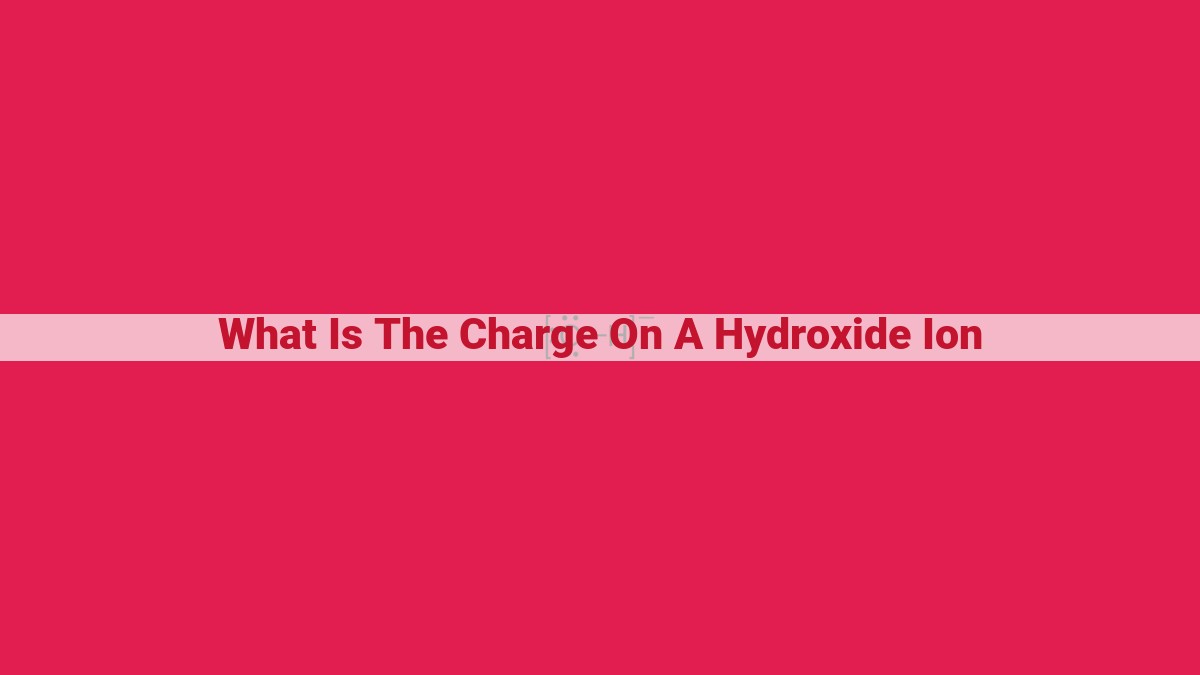
The hydroxide ion has a negative charge of -1. This is due to its structure, which consists of an oxygen atom bonded to a hydrogen atom. The oxygen atom has eight valence electrons, while the hydrogen atom has one. When the hydrogen atom loses its valence electron, it becomes a hydrogen ion with a charge of +1. The oxygen atom then gains the electron, becoming a hydroxide ion with a charge of -1.
The Fascinating World of Atomic Structure: Unveiling the Building Blocks of Matter
At the very heart of every object, from the towering mountains to the tiniest microorganisms, lies a realm of extraordinary complexity and wonder—the atomic world. In this realm, matter is composed of tiny particles called atoms, the fundamental building blocks of our universe.
Unveiling the Atom
Imagine an atom as a miniature solar system. At the center, you have a nucleus, a dense core made up of positively charged particles called protons and uncharged particles called neutrons. Surrounding this nucleus is a dynamic cloud of negatively charged particles known as electrons.
Electrons are arranged in shells or energy levels. The outermost shell, known as the valence shell, is particularly important in chemical reactions. These valence electrons determine the atom’s ability to bond with other atoms, forming the molecules that make up everything around us.
Digging Deeper: The Nucleus and Atomic Identity
Protons play a crucial role in determining an element’s identity and atomic number. Each element has a unique atomic number, which represents the number of protons in its nucleus. This number defines the element’s position on the periodic table and its chemical properties.
Neutrons and the Mass of the Atom
While protons contribute to the atom’s positive charge, neutrons add to its mass without affecting the charge. The sum of protons and neutrons in the nucleus is called the mass number. However, atoms of the same element can have different numbers of neutrons, resulting in different isotopes with slightly varying masses.
This concept of isotopes has significant implications in various fields, such as nuclear power and medicine.
Atomic Mass and the Mystery of Isobars
The atomic mass of an element is an average of the masses of all its isotopes, weighted by their abundance. However, sometimes atoms with different atomic numbers can have the same mass number. These atoms are called isobars and provide intriguing insights into the intricacies of atomic structure.
Protons and Atomic Number: The Identity of Elements
In the vast realm of atoms, protons hold the key to unlocking an element’s unique identity. These powerful particles reside in the nucleus, the central core of an atom. Their presence determines not only an element’s atomic number but also its fundamental nature.
Each element is assigned a unique atomic number, a numerical value that corresponds to the number of protons it possesses. This number is like an element’s personal identification code, distinguishing it from all others. For instance, hydrogen has an atomic number of 1, carbon 6, and gold 79. This simple yet crucial number reveals the arrangement of electrons in an atom’s outer orbit, which in turn dictates the element’s chemical behavior.
Calculating an atomic number is a straightforward task. Simply count the number of protons in an atom’s nucleus, and you have the atomic number. This number remains constant for a given element, regardless of the number of neutrons or electrons present. It is this unchanging identity that allows us to classify elements into the familiar periodic table, arranging them in order of increasing atomic number.
So, the next time you encounter an atom, remember the significance of its protons. They are the tiny gatekeepers of atomic identity, determining an element’s unique place in the tapestry of the universe.
Neutrons and Mass Number: The Heart of Atomic Identity
In the realm of the atom, where the tiniest building blocks of our universe reside, the presence of neutrons plays a crucial role in shaping an atom’s identity and behavior. Neutrons, the uncharged companions of protons, reside within the atom’s nucleus, alongside their positively charged counterparts. Their presence, or rather the lack thereof, can alter the atom’s mass number and give rise to the fascinating phenomenon of isotopes.
The mass number of an atom represents the total number of protons and neutrons it possesses. It is a fundamental characteristic that distinguishes one element from another. While protons determine the atomic number (the unique fingerprint of an element), neutrons contribute to the overall mass of the atom.
Isotopes are thrilling variants of an element that share the same atomic number but differ in their mass number. This difference stems from the varying number of neutrons they harbor. Despite their differing masses, isotopes behave chemically in very similar ways, as they still hold the same number of electrons (the chemical workhorses).
For instance, the element carbon possesses three naturally occurring isotopes: carbon-12, carbon-13, and carbon-14. While carbon-12 contains six protons and six neutrons, carbon-13 has six protons and seven neutrons, and carbon-14 boasts six protons but eight neutrons. These isotopes share the same chemical properties but find distinct applications in fields such as medicine (carbon-14 dating) and nuclear chemistry.
The presence of neutrons not only influences an atom’s mass but also governs its stability. Atoms with an uneven number of neutrons may exhibit radioactive behavior, releasing energy in the form of radiation to achieve a more stable configuration. This process forms the basis of nuclear reactions and provides valuable insights into the inner workings of matter.
In the grand tapestry of atomic structure, neutrons play a pivotal role in defining mass number and fostering the fascinating world of isotopes. Their presence shapes the properties of elements and opens up avenues for scientific exploration. Understanding the significance of neutrons is a testament to the intricate and captivating nature of the atomic realm.
Atomic Mass and Isotopes: Unraveling the Mass Puzzle
In the realm of chemistry, the atomic mass of an element holds a profound significance. It represents the average mass of all naturally occurring isotopes of that element, weighted by their relative abundances. This value, expressed in atomic mass units (amu), provides crucial insights into an element’s composition and its behavior in chemical reactions.
The key to understanding atomic mass lies in isotopes. Isotopes are atoms of the same element that possess an identical number of protons (atomic number) but differ in the number of neutrons. Think of isotopes as siblings of the same element, sharing the same family traits (atomic number) but with varying weights (neutron count).
For instance, carbon has three naturally occurring isotopes:
- Carbon-12 (6 protons, 6 neutrons)
- Carbon-13 (6 protons, 7 neutrons)
- Carbon-14 (6 protons, 8 neutrons)
Each isotope contributes to the element’s atomic mass, with the relative abundance of each isotope influencing the average value. Carbon-12, the most abundant isotope, has an atomic mass of 12 amu. Carbon-13 and Carbon-14 have atomic masses of 13 amu and 14 amu, respectively.
Additionally, there’s a fascinating concept known as isobars. These are atoms of different elements that share the same mass number. Isobars may have different atomic numbers but end up with the same total number of protons and neutrons. For example, Potassium-40 and Argon-40 are isobars, both with 40 nucleons.
An intriguing aspect of isotopes is their applications. Carbon-14, a radioactive isotope, finds use in carbon dating, a technique that determines the age of ancient artifacts and fossils. Understanding the atomic mass and isotope composition of elements is crucial for scientists in various fields, including chemistry, physics, and geology.
In summary, atomic mass provides a valuable measure of an element’s average weight, and isotopes, with their varying neutron counts, contribute to this value. Isobars add another dimension to atomic structure, highlighting the diversity within the periodic table. Understanding these concepts is essential for comprehending the fundamental building blocks of matter and the intricacies of their interactions.
Atoms with Electric Charges: Ions
Have you ever wondered how atoms can gain a magical ability to carry electric charges? Meet ions, the fascinating particles that bring life to chemical reactions and shape the world around us.
Formation of Ions: A Chemical Transformation
Imagine an atom as a tiny universe, with a dense nucleus at its core and a cloud of electrons orbiting around it. In the world of atoms, electrons are the key players in determining an atom’s behavior. When an electron decides to leave its comfortable abode and venture out into the unknown, the atom goes through a remarkable transformation. It becomes an ion.
Anions and Cations: The Positively and Negatively Charged Ions
Just like there are two sides to every story, there are also two main types of ions: anions and cations. Anions, the rebels of the ion world, have a surplus of electrons, giving them a negative charge. Cations, on the other hand, are the atom’s version of superheroes, with a positive charge due to a shortage of electrons.
The Significance of Ions: A Dance of Charged Particles
Ions are not just charged loners; they play a crucial role in the vibrant symphony of chemical reactions. They can attract or repel each other, forming bonds that lead to the creation of molecules, compounds, and even the structures of living organisms. Without ions, our world would be a dull and inert place.
The world of ions is a testament to the incredible diversity and complexity of the atomic realm. From their formation to their interactions, ions drive the chemical reactions that shape our planet and make life possible. Understanding their nature is not just a scientific pursuit; it’s a journey into the fundamental building blocks of our universe.
Electronegativity and Electron Affinity: The Interplay of Atoms
In the intricate world of atoms, there exists a delicate balance between their ability to attract and hold onto electrons. This captivating dance of electrons is governed by two fundamental properties: electronegativity and electron affinity. Comprehending these concepts is akin to unlocking the secret code that underlies chemical bonding and the behavior of elements.
Electronegativity: The Pull of the Nucleus
Electronegativity measures the tendency of an atom to attract electrons towards its nucleus. This invisible force arises from the positive charge of the nucleus, which exerts a gravitational pull on the negatively charged electrons. The greater the electronegativity, the stronger the nucleus’s grip on its electrons.
Electron Affinity: The Eagerness to Embrace Electrons
Electron affinity, on the other hand, quantifies the eagerness of an atom to gain electrons. It represents the energy released when an atom accepts an additional electron. Atoms with high electron affinities have a strong desire to complete their electron shells, making them highly reactive in forming chemical bonds.
The Dance of Electrons: Electronegativity and Electron Affinity in Action
The interplay of electronegativity and electron affinity determines the type and strength of chemical bonds formed between atoms. When atoms with high electronegativity bond with atoms with low electronegativity, the electrons shift towards the more electronegative atom. This unequal distribution of electrons creates a polar covalent bond, where one atom has a partial negative charge and the other has a partial positive charge.
In contrast, atoms with similar electronegativities tend to form nonpolar covalent bonds, where the electrons are equally shared between the atoms. The presence of lone pairs of electrons, which are not involved in bonding, also influences the polarity of molecules.
Delving into the fascinating world of atomic structure provides invaluable insights into the behavior of elements and chemical reactions. By understanding the concepts of electronegativity and electron affinity, we gain a deeper appreciation for the intricate dance of electrons that governs the formation of the molecules that make up our universe.
Ionization Energy: The Key to Understanding Chemical Reactions
In the captivating realm of chemistry, ionization energy holds a pivotal position, unlocking the secrets of chemical reactions and the behavior of atoms. It represents the energy required to remove an electron from an atom or ion.
Determining the ionization energy of an atom is crucial because it influences its chemical reactivity. Atoms with low ionization energies readily donate electrons, forming positive ions. Conversely, atoms with high ionization energies resist losing electrons, preferring to form negative ions.
Numerous factors shape the ionization energy of an atom. One is atomic size. The larger the atom, the further its electrons are from the nucleus, resulting in lower ionization energy. Think of it as trying to pull a ball from a deep hole versus a shallow one.
Another factor is nuclear charge. The more protons an atom has, the stronger the electrostatic attraction between the nucleus and electrons, leading to higher ionization energy. It’s like trying to pull a magnet away from a metal surface – the stronger the magnet, the harder it is.
The position of an electron in an atom’s electron configuration also influences ionization energy. Core electrons, closer to the nucleus, experience greater attraction and higher ionization energies. Valence electrons, on the other hand, are more loosely held and have lower ionization energies.
Understanding ionization energy is essential for predicting the chemical behavior of elements. Elements with low ionization energies are highly reactive, forming ionic compounds, while elements with high ionization energies tend to form covalent compounds. This concept forms the foundation of our understanding of chemical bonding and the myriad properties of the elements that make up our world.