Hydration Shells: Understanding The Interactions And Effects In Aqueous Solutions
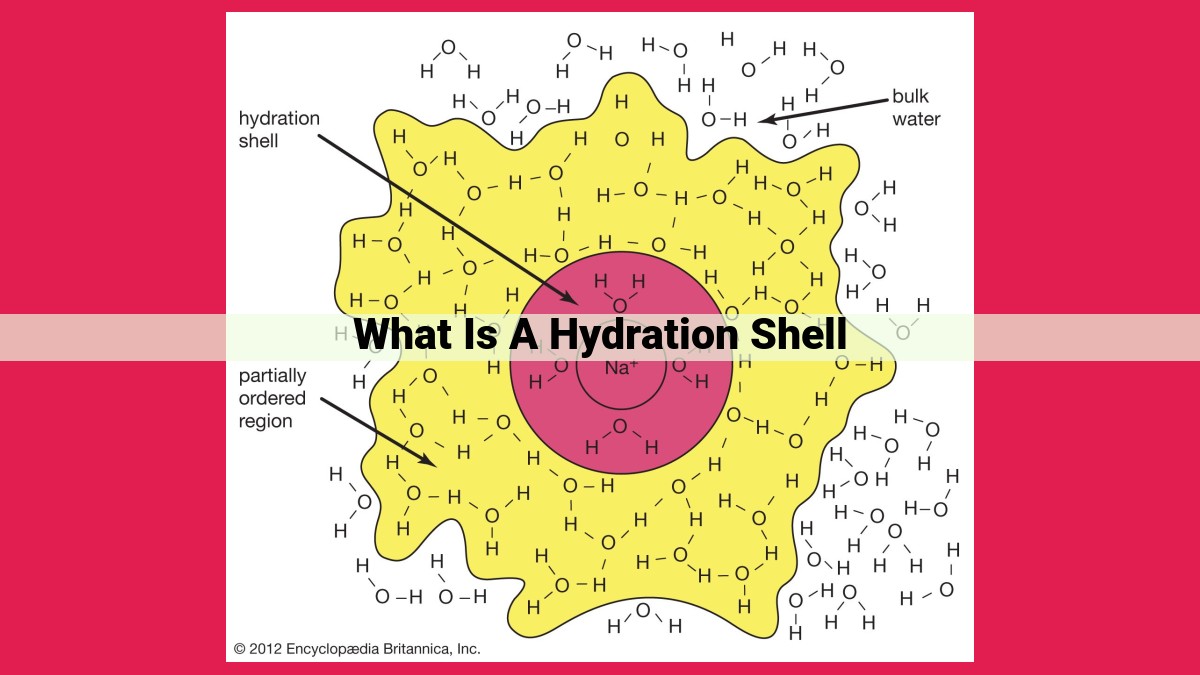
A hydration shell is a layer of water molecules surrounding ions or polar molecules in a solution. It forms through interactions such as hydrogen bonding and ion-dipole forces, creating a stable arrangement of water molecules around the solute. The hydration number, influenced by ion-dipole interactions and electrostatic effects, indicates the number of water molecules directly bound to the solute. The energy associated with hydration, including electrostatic and van der Waals interactions, affects the solute’s stability and solubility in water.
Understanding Hydration Shells
In the realm of chemistry, the concept of hydration shells plays a crucial role in understanding solution behavior. When a solute dissolves in a solvent, it becomes surrounded by a sphere of solvent molecules known as a hydration shell. This phenomenon, known as solvation, is fundamental to numerous chemical processes.
Solvation encompasses different types, including ion-dipole interactions and hydrogen bonding. Ion-dipole interactions occur when an ion interacts with a neutral molecule having a polar covalent bond, resulting in electrostatic attraction or repulsion. Hydrogen bonding is a strong dipole-dipole interaction that occurs between a hydrogen atom bonded to a highly electronegative atom and another electronegative atom, such as oxygen, nitrogen, or fluorine.
These interactions are key in the formation of hydration shells. Water molecules, with their polar nature and ability to form hydrogen bonds, are particularly effective at solvating ions and polar molecules. The strength of these interactions determines the extent of solvation and the stability of the hydration shell.
Understanding Hydrogen Bonding in Hydration Shells
Immerse yourself in the intricate realm of hydrogen bonding—the enigmatic force that shapes our world on the molecular level. In the context of hydration shells, these bonds play a pivotal role, endowing water molecules with a remarkable ability to surround and stabilize ions or other polar solutes.
Unveiling the Nature of Hydrogen Bonds
At their core, hydrogen bonds are intermolecular interactions that arise when a hydrogen atom is covalently bonded to an electronegative atom, such as nitrogen, oxygen, or fluorine. This creates a polar covalent bond, with the hydrogen atom carrying a partial positive charge and the electronegative atom carrying a partial negative charge.
Hydrogen Bonding’s Role in Solvation and Hydration Shells
The partial charges inherent to hydrogen bonds allow water molecules to interact with each other and with other polar molecules. It’s like a molecular dance, where hydrogen atoms from one water molecule are attracted to the oxygen atoms of neighboring water molecules. These interactions form an interconnecting network of hydrogen bonds, creating a cohesive hydration shell around ions or other charged species.
Hydrogen Bonding’s Influence on Secondary Hydration Shells
The influence of hydrogen bonding extends beyond the primary hydration shell. Water molecules can form additional hydrogen bonds with water molecules in the secondary hydration shell, which surrounds the primary shell. These secondary hydration shells contribute to the solubility of ions and impact their transport properties, such as diffusion and viscosity.
Ion-Dipole Interactions: The Key Players in Hydration Shell Formation
Imagine water molecules as tiny magnets, with slightly positive regions (hydrogens) and slightly negative regions (oxygens). When an ion (an electrically charged atom or molecule) enters the water, these water magnets dance around it. This dance is driven by ion-dipole interactions, a special type of force that occurs between charged particles and polar molecules like water.
Positive ions attract the negative ends of water molecules, while negative ions attract the positive ends. This attraction leads to the formation of a hydration shell, a layer of water molecules that surround the ion like a protective bubble. The strength of the ion-dipole interaction determines how tightly the water molecules bind to the ion, which in turn affects the hydration number.
The hydration number represents the average number of water molecules directly attached to an ion. Ions with stronger ion-dipole interactions have higher hydration numbers because water molecules cling to them more tightly. For example, sodium ions (Na+) have a hydration number of about six, while calcium ions (Ca2+) have a hydration number of about eight due to their higher charge and stronger attraction to water molecules.
Ion-dipole interactions are crucial for understanding the behavior of ions in water. They influence how ions dissolve, move, and interact with each other. By studying these interactions, scientists can gain insights into the properties of materials and biological systems.
Hydration Number
- Definition and importance of hydration number
- Factors affecting hydration number, such as ion-dipole interactions and electrostatic effects
- Implications for hydration shell structure
Hydration Number: A Measure of Ion Surroundings
In the fascinating realm of chemistry, the hydration number stands out as a crucial concept that quantifies the number of water molecules closely associated with an ion in a solution. This number holds great significance in understanding the behavior of ions in aqueous environments.
Factors influencing the hydration number are multifaceted. Ion-dipole interactions, which exist between the ion’s charge and the water molecule’s polar nature, serve as a primary driving force. Ions with stronger charges tend to attract more water molecules, resulting in a higher hydration number.
Electrostatic effects also play a role. As ions approach each other, electrostatic repulsion can cause the hydration shells to shrink, reducing the hydration number. Conversely, ions with opposite charges attract one another, enhancing hydration numbers due to the formation of extended hydration shells.
The hydration number has profound implications for the structure of hydration shells. Ions with a low hydration number often reside in primary hydration shells, where water molecules are directly coordinated to the ion. In contrast, ions with high hydration numbers form extended hydration shells, consisting of multiple layers of water molecules surrounding the ion.
Understanding the hydration number is essential in various scientific disciplines, from chemistry and biology to environmental science. It controls the solubility, reactivity, and transport of ions in aqueous systems, affecting processes ranging from biological metabolism to water purification technologies. By unraveling the complexities of hydration numbers, scientists gain invaluable insights into the behavior of ions in the world around us.
Electrostatic Interactions: Shaping Hydration Shells
When ions or polar molecules dissolve in water, they become surrounded by a cloud of water molecules called a hydration shell. These shells form due to the electrostatic interactions between the dissolved species and water molecules.
Overview of Electrostatic Forces
Electrostatic interactions are forces that arise between charged particles. In the context of hydration, these forces exist between charged ions or polar molecules and the polar water molecules. Water molecules have a partially positive charge on one end and a partially negative charge on the other.
Role in Solvation and Hydration Shells
Electrostatic interactions play a crucial role in solvation, the process by which ions or molecules dissolve in a solvent. These interactions attract the solvent molecules to the dissolved species, forming a hydration shell around them. The strength of the electrostatic interactions determines the thickness and stability of the hydration shell.
Relationship with Ionic Strength and Hydration Number
The ionic strength of a solution is a measure of the concentration of ions in the solution. It influences the hydration number of ions, which is the average number of water molecules in their first hydration shell. Higher ionic strength weakens the electrostatic interactions between ions and water molecules, reducing the hydration number. This is because the high concentration of ions creates competition for the water molecules.
Electrostatic interactions are essential in shaping hydration shells. They determine the structure, thickness, and stability of these shells, which are crucial for the behavior of ions and molecules in aqueous solutions. Understanding these interactions helps us unravel the intricacies of solvation and its implications for chemical reactions and biological processes.
Hydration Energy: The Driving Force Behind Solvation
In the realm of chemistry, understanding the forces that govern the interactions between molecules is crucial. When an ion or a polar molecule dissolves in a solvent, it becomes surrounded by a shell of solvent molecules. This entourage of solvent molecules is known as the hydration shell.
The formation of a hydration shell is driven by a phenomenon called hydration energy. Hydration energy is the energy released when an ion or a polar molecule interacts with water molecules. This energy is derived from the various forces that exist between the solute and the solvent.
One of the primary forces contributing to hydration energy is electrostatic interactions. These forces act between charged ions and polar molecules. When an ion dissolves in water, it creates an electrostatic field that attracts water molecules. The positively charged ions attract negatively charged water molecules, while negatively charged ions attract positively charged water molecules.
Another force that influences hydration energy is van der Waals interactions. These interactions are weak attractive forces that exist between all molecules. In the context of hydration, van der Waals interactions contribute to the cohesion of water molecules around the solute.
The combination of electrostatic and van der Waals interactions creates a cohesive force that binds water molecules to the solute, forming a stable hydration shell. The strength of the hydration shell depends on the magnitude of these interactions.
The hydration energy of an ion or a polar molecule directly impacts its behavior in solution. Ions with a high hydration energy are more strongly bound to water molecules and have a lower tendency to dissociate. This property influences the solubility, reactivity, and transport of the ion in aqueous solutions.
In conclusion, hydration energy is a fundamental concept in chemistry that explains the interactions between ions or polar molecules and water molecules. Understanding hydration energy is essential for deciphering the behavior of molecules in aqueous solutions and plays a crucial role in various chemical and biological processes.
Entropy Changes in Hydration Processes
At the core of hydration lies a delicate interplay of order and disorder, encapsulated by the concept of entropy. Entropy measures the degree of disorder within a system, and in hydration, it reigns supreme.
Two opposing forces govern entropy changes in hydration:
- Polarity: Hydrophilic groups, such as those containing hydroxyl (-OH) or carbonyl (C=O) bonds, have a strong affinity for water and promote order, decreasing entropy.
- Nonpolarity: Hydrophobic groups, like hydrocarbons, repel water and induce disorder, increasing entropy.
Hydration Shells
Around ions or polar molecules, a structured layer of water molecules forms, known as a hydration shell. The strength of this shell depends on the balance between hydrophilic and hydrophobic interactions.
- Hydrophilic Ions or Molecules: Water molecules align themselves around hydrophilic groups, forming strong hydration shells. This orderliness reduces the system’s entropy.
- Hydrophobic Ions or Molecules: Nonpolar groups disrupt the hydration shell, creating disorder and increasing entropy.
Solvation
Entropy also plays a crucial role in the process of solvation, where a solute dissolves in a solvent.
- Hydrophilic Solutes: Hydrophilic solutes form strong hydration shells, which reduces the system’s overall entropy.
- Hydrophobic Solutes: Nonpolar solutes break up hydration shells, increasing entropy.
Understanding entropy changes in hydration not only sheds light on the behavior of solutes in solution but also has implications for biological processes, where hydration plays a vital role in protein folding and the stability of biological membranes.
Van der Waals Interactions: The Invisible Forces in Hydration
In the realm of chemistry, the microscopic interactions between molecules and ions play a crucial role in determining their properties and behavior. Among these interactions, van der Waals forces emerge as an often-overlooked, but essential player in the formation and dynamics of hydration shells.
Understanding van der Waals Forces
Van der Waals forces are intermolecular forces that arise from the temporary fluctuations in electron density around atoms and molecules. These forces are weaker than covalent or ionic bonds but play a significant role in various physical phenomena, including solvation and hydration.
Role in Solvation and Hydration Shells
When a solute (a substance being dissolved) interacts with a solvent (such as water), solvent molecules form a hydration shell around the solute. Van der Waals forces contribute to the stability of this hydration shell by providing dispersion forces between the solute and solvent molecules. Dispersion forces arise from the instantaneous polarization of molecules, creating attractive forces between them.
Significance in Hydrophobic Interactions
Hydrophobic interactions refer to the tendency of nonpolar molecules (molecules without a permanent dipole moment) to associate in water. Van der Waals forces play a crucial role in these interactions, as they provide cohesive forces between the nonpolar molecules. These forces help exclude water molecules from the vicinity of the nonpolar molecules, leading to the formation of hydrophobic clusters.
While van der Waals forces may seem subtle, their cumulative effect significantly influences the structure and stability of hydration shells. Understanding these forces is essential for comprehending the behavior of molecules in solution, the solubility of substances, and various biological processes that rely on the interactions of water with molecules and ions. By appreciating the role of van der Waals forces, we gain a deeper understanding of the intricate world of molecular interactions and their impact on our macroscopic observations.
Kirkwood-Buff Theory: Unveiling the Intricate World of Hydration Shells
In the realm of chemistry, liquids intertwine with solutes, forming intricate dance of interactions that shape the very nature of solutions. Hydration shells, layers of solvent molecules that surround dissolved ions or molecules, play a central role in this captivating world. The Kirkwood-Buff theory provides a profound framework for understanding these hydration shells, shedding light on their structure and interactions.
Understanding the Kirkwood-Buff Theory
The Kirkwood-Buff theory delves into the relationship between electrostatic interactions and the structure of hydration shells. It postulates that electrostatic forces between dissolved ions or molecules and water molecules govern the number and arrangement of water molecules within the hydration shell.
Impact of Electrostatic Interactions
Electrostatic interactions, the invisible forces between charged particles, play a crucial role in the formation and stability of hydration shells. Positively charged ions attract water molecules with their negative partial charges, forming a tightly bound inner hydration shell. The hydration number, representing the number of water molecules in the inner hydration shell, is influenced by the strength of these electrostatic interactions.
Ionic Strength and Hydration Shell Structure
The theory further reveals the influence of ionic strength on hydration shell structure. Ionic strength, a measure of the concentration of ions in solution, affects the electrostatic forces between ions and water molecules. High ionic strength weakens these forces, leading to a decrease in hydration number and a more diffuse hydration shell.
Implications for Hydration Shell Structure
The Kirkwood-Buff theory provides valuable insights into the structural organization of hydration shells. It helps explain the variations in hydration number observed for different ions and molecules, and elucidates the dynamic nature of hydration shells as they adapt to changing ionic strength. This knowledge is essential for comprehending the behavior of ions and molecules in aqueous solutions, paving the way for advancements in diverse fields such as electrochemistry, biochemistry, and environmental science.