Understanding Heat Of Reaction: Essential Concepts For Chemical Reactions
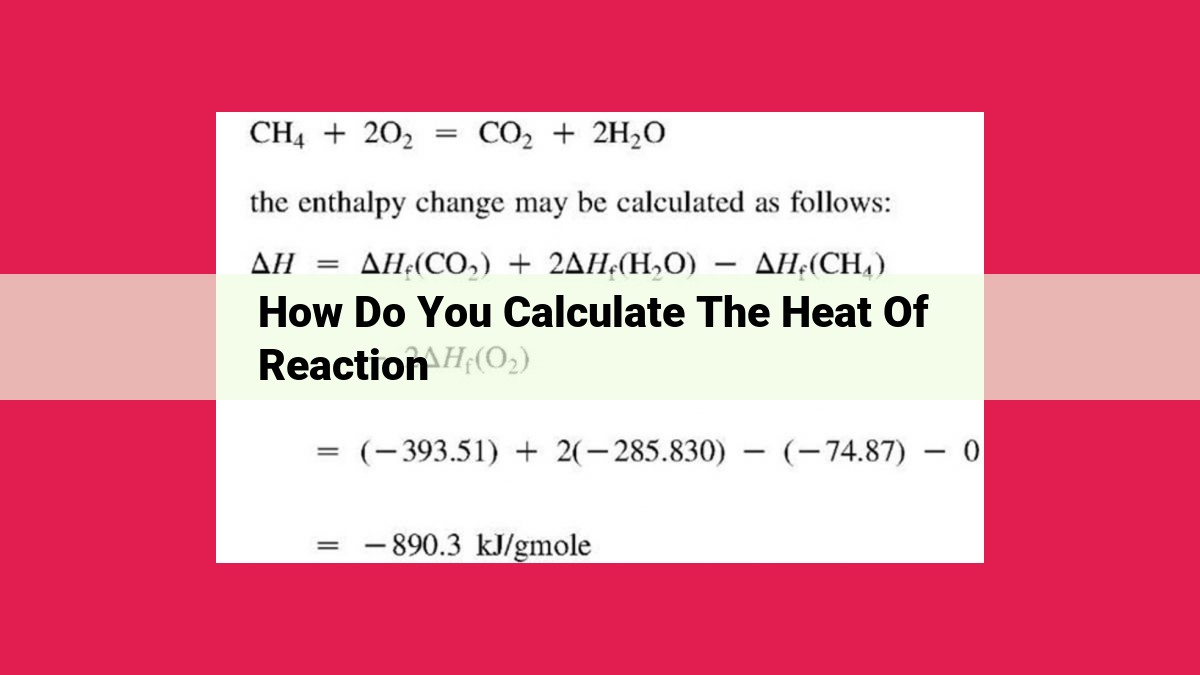
The heat of reaction, a crucial parameter in chemistry, represents the energy change associated with a chemical reaction. It is typically calculated by determining the enthalpy change (ΔH), which is the difference in energy content between the reactants and products. One key method is Hess’s Law, which allows for the calculation of enthalpy changes by combining thermochemical equations with known enthalpy values for individual reactions. Additionally, standard enthalpies of formation, which represent the enthalpy changes during the formation of compounds from their constituent elements, are used to simplify calculations. Thermochemical equations provide a convenient way to express enthalpy changes directly in the equation. Furthermore, bond energies and calorimetry techniques can be employed to estimate heat changes, while heat capacity plays a role in understanding the temperature changes associated with reactions.
The Vital Importance of Calculating Heat of Reaction in Chemistry
In the captivating realm of chemistry, understanding the heat of reaction is paramount, akin to unraveling the secrets of an enigmatic language. It unveils the intricate dance of energy within chemical transformations, illuminating the path to countless discoveries.
Chemical reactions, like miniature fireworks, release or absorb energy in the form of heat. Calculating this heat of reaction is akin to a detective analyzing the energetic fingerprint of a reaction, revealing its hidden powers and propensity for change. By unraveling this enigmatic code, chemists gain the ability to craft novel materials, engineer sustainable processes, and decipher the intricate workings of the natural world.
Moreover, the calculation of heat of reaction unlocks a treasure trove of insights into the enthalpy of reaction, a fundamental property that measures the energy released or absorbed during a chemical transformation. Enthalpy change, symbolized by the Greek letter delta (Δ), is the governing force behind reactions, dictating whether they will proceed spontaneously or require external energy input.
Armed with the knowledge of heat of reaction and enthalpy change, chemists become alchemists, wielding the power to manipulate and control chemical transformations. They can forge materials with tailored properties, harness energy from chemical reactions, and even predict the outcome of complex chemical systems.
Calculating the Heat of Reaction: Understanding the Energy Changes in Chemical Reactions
In chemistry, understanding the energy changes that occur during reactions is crucial. One key aspect is calculating the heat of reaction, which reveals the amount of energy released or absorbed in a chemical process. This information plays a vital role in predicting reaction outcomes, designing experiments, and optimizing industrial processes.
Definition and Significance of Enthalpy of Reaction
The enthalpy of reaction (ΔH) is a measure of the heat change that accompanies a chemical reaction. It represents the difference in the total enthalpy of the reactants and the products. Enthalpy is a thermodynamic property that incorporates both the internal energy of a system and its pressure-volume work. Understanding ΔH allows us to determine whether a reaction is exothermic, releasing energy, or endothermic, absorbing energy.
Example:
Consider the reaction of burning methane (CH₄) with oxygen (O₂):
CH₄ + 2O₂ → CO₂ + 2H₂O + energy
This reaction is exothermic, meaning it releases heat. The negative sign of ΔH in the thermochemical equation indicates that energy is lost from the system.
Calculating the Enthalpy of Reaction: Unraveling the Secrets of Chemical Heat
In the realm of chemistry, understanding the heat of reaction is paramount. It tells us the amount of energy absorbed or released when chemical bonds are formed or broken. This knowledge empowers chemists to predict reaction outcomes, design new materials, and comprehend numerous phenomena.
One of the most potent tools for calculating enthalpy changes is Hess’s law. Hess’s law states that the enthalpy change for a chemical reaction is independent of the pathway taken. This means we can break down complex reactions into simpler steps and add their enthalpy changes to obtain the overall enthalpy change. It’s like navigating a maze: we don’t have to traverse every path; we can take shortcuts!
Using Hess’s law involves a few simple steps:
-
Identify the overall reaction: Determine the chemical equation that represents the reaction of interest.
-
Break down into simpler steps: Divide the overall reaction into smaller steps, each representing a well-known enthalpy change.
-
Reverse and multiply steps: If necessary, reverse any steps that are not in the same direction as the overall reaction and adjust their enthalpy changes accordingly.
-
Add up the enthalpy changes: Sum the enthalpy changes of all the steps to obtain the overall enthalpy change.
Hess’s law has revolutionized enthalpy calculations, simplifying what was once a laborious process. It’s a key tool for chemists, enabling them to solve complex problems with ease.
Mastering Hess’s Law: A Step-by-Step Guide
Calculating the heat of reaction is crucial in chemistry, revealing the energy changes that occur during chemical transformations. Hess’s law serves as a powerful tool for unraveling these energy changes, and understanding its steps is essential for solving complex thermodynamics problems.
1. Identify the Target Reaction:
Begin by defining the target reaction you wish to calculate the enthalpy change for. Carefully balance the equation to ensure the number of atoms of each element is equal on both sides.
2. Break Down into Simpler Reactions:
Hess’s law allows us to break down the target reaction into a series of simpler reactions with known enthalpy changes. Each step must result in the production of one or more of the products in the target reaction.
3. Reverse and Multiply Reactions:
IfNeeded, reverse or multiply the simpler reactions to align their products with the reactants or products in the target reaction. Adjust the enthalpy changes of these modified reactions accordingly.
4. Sum Enthalpy Changes:
Finally, sum the enthalpy changes from all the simpler reactions. The overall enthalpy change will be equal to the algebraic sum of these enthalpy changes.
Example:
Let’s calculate the enthalpy change for the combustion of methane:
CH₄(g) + 2O₂(g) → CO₂(g) + 2H₂O(l)
Simpler Reactions:
- CH₄(g) + O₂(g) → CO(g) + 2H₂O(l) ΔH = -802 kJ
- CO(g) + 0.5O₂(g) → CO₂(g) ΔH = -283 kJ
Solving:
- Reverse the second reaction and multiply by 2: 2CO₂(g) + O₂(g) → 2CO(g) ΔH = 566 kJ
- Sum the enthalpy changes: -802 kJ + 566 kJ = -236 kJ
Therefore, the combustion of methane releases 236 kJ of heat.
Unlocking the Secrets of Enthalpy: A Guide to Calculating the Heat of Reaction
In the realm of chemistry, the ability to calculate the heat of reaction is a cornerstone for understanding chemical processes and designing new materials. This concept encompasses the energy changes that occur during a chemical reaction, and it holds profound significance in various scientific fields.
One key concept in this endeavor is the standard enthalpy of formation. This parameter, represented by the symbol ΔHf°, quantifies the enthalpy change associated with the formation of 1 mole of a compound from its constituent elements. It is a fundamental thermodynamic property that provides insights into the stability and reactivity of molecules.
The standard enthalpy of formation is negative for stable compounds, indicating the release of energy during their formation. Conversely, a positive ΔHf° denotes an endothermic reaction, which requires energy input to proceed. By tabulating these values for a wide range of compounds, chemists have created a valuable resource for calculating enthalpy changes in various chemical processes.
Example:
Consider the combustion of methane:
CH₄(g) + 2O₂(g) → CO₂(g) + 2H₂O(l) ΔHf° = -890.4 kJ/mol
The negative ΔHf° value for this reaction indicates that the formation of carbon dioxide and water from their elements releases energy. This energy is liberated as heat, making the combustion of methane an exothermic process.
Calculating the Enthalpy of Reaction: Unraveling the Secrets of Heat Exchange
In the realm of chemistry, understanding the heat of reaction is paramount to unriddle the mysteries of chemical transformations. This elusive quantity, denoted as enthalpy change, measures the heat absorbed or released during a chemical reaction. It serves as a crucial tool for predicting reaction spontaneity, evaluating reaction pathways, and designing efficient industrial processes.
One of the most versatile techniques for calculating enthalpy changes is Hess’s law. This powerful rule allows us to deduce the enthalpy change of a complex reaction by combining simpler reactions for which the enthalpy change is known. Imagine a culinary masterpiece: by understanding the flavors and textures of individual ingredients, we can confidently predict the exquisite taste of the final dish. Hess’s law empowers us with a similar predictive ability in the chemical realm.
Standard enthalpy of formation (ΔHf°) plays a pivotal role in this endeavor. It measures the enthalpy change when one mole of a compound is formed from its constituent elements in their standard states. Armed with this knowledge, we can calculate the enthalpy change of any reaction by considering the enthalpy of formation of the reactants and products.
For instance, let’s calculate the enthalpy change for the combustion of methane (CH4):
CH4 + 2 O2 → CO2 + 2 H2O
Using tabulated values of ΔHf° for each species:
ΔHf°(CH4) = -74.8 kJ/mol
ΔHf°(O2) = 0 kJ/mol
ΔHf°(CO2) = -393.5 kJ/mol
ΔHf°(H2O) = -285.8 kJ/mol
ΔH° = ΣΔHf°(products) – ΣΔHf°(reactants)
ΔH° = [-393.5 kJ/mol + (2)(-285.8 kJ/mol)] – [-74.8 kJ/mol + (2)(0 kJ/mol)]
ΔH° = -890.3 kJ/mol
This negative value for ΔH° indicates that the combustion of methane is an exothermic reaction, releasing 890.3 kJ of heat per mole of methane consumed.
Calculating the Heat of Reaction: Unraveling the Energetics of Chemical Change
In the vast realm of chemistry, understanding the energetics of reactions is crucial. Calculating the heat of reaction provides invaluable insights into the energy changes accompanying chemical transformations, guiding our knowledge of chemical processes.
Hess’s Law: A Framework for Enthalpy Calculations
Enthalpy, symbolized as H, represents the total thermal energy of a system. Hess’s law offers a powerful tool to determine enthalpy changes (ΔH) in a reaction by combining known enthalpy changes from smaller, simpler reactions. It’s like piecing together a puzzle, where individual reactions serve as building blocks to calculate the overall enthalpy change.
Standard Enthalpy of Formation: A Reference Point for Energy
Every compound has a standard enthalpy of formation (ΔHf°), which represents the energy change associated with the formation of one mole of that compound from its constituent elements in their standard states. By knowing the standard enthalpies of formation of reactants and products, we can calculate the enthalpy change for any reaction.
Thermochemical Equations: The Energetic Language of Chemistry
Thermochemical equations are a language that describes chemical reactions in terms of energy changes. They include ΔH values as coefficients in front of reaction components, allowing us to determine the heat absorbed or released during a reaction.
Bond Energy and Calorimetry: Measuring the Heat of Reaction
Bond energy, the energy required to break a chemical bond, plays a significant role in heat of reaction calculations. Calorimetry, a technique that measures heat changes in reactions, provides experimental data for calculating bond energies and other thermodynamic properties.
Heat Capacity: Predicting Temperature Changes
Heat capacity, a measure of the amount of heat required to raise the temperature of a substance by one degree Celsius, helps us predict temperature changes during reactions. It enables us to understand how reactions will affect the temperature of their surroundings, whether they will release heat (exothermic) or absorb heat (endothermic).
Understanding the Secrets of Heat of Reaction
In the captivating world of chemistry, understanding the heat of reaction is akin to deciphering a secret code, revealing the energy changes that drive chemical transformations. Enthalpy of reaction is the key to unlocking this cryptic knowledge, representing the total energy absorbed or released during a chemical process.
Hess’s law emerges as a powerful tool, granting us the ability to calculate enthalpy changes even for complex reactions. This ingenious law states that the enthalpy change for a reaction is equal to the sum of the enthalpy changes of the individual steps leading to that reaction. By strategically combining these steps, we can unravel the energy secrets of any reaction.
Adding another layer of complexity to this chemical puzzle, standard enthalpy of formation emerges as a crucial concept. It represents the enthalpy change when one mole of a compound is formed from its constituent elements in their standard states. Armed with this knowledge, we can utilize standard enthalpies of formation to determine the enthalpy change for any given reaction.
Thermochemical equations paint a vivid picture of chemical reactions, depicting the energy changes associated with the formation of reactants and products. These equations provide a direct pathway for calculating enthalpy changes, guiding us through the labyrinth of complex chemical transformations.
Delving further into the intricacies of heat of reaction, bond energy reveals its pivotal role. Every chemical bond possesses a specific amount of energy, and when bonds are broken or formed, this energy is either absorbed or released. By meticulously accounting for bond energies, we can accurately gauge the heat of reaction.
Calorimetry emerges as an invaluable experimental technique, allowing us to measure the heat changes that occur during chemical reactions. This precise method empowers us to quantify the energy absorbed or released, providing empirical evidence for our theoretical calculations.
Finally, the enigmatic concept of heat capacity enters the stage, representing the amount of heat required to raise the temperature of a substance by one degree. This property plays a significant role in predicting temperature changes during reactions, ensuring that we fully comprehend the energetic dance that unfolds within chemical transformations.
Unlocking the Secrets of Heat of Reaction: A Journey through Bond Energy
In the realm of chemistry, the heat of reaction plays a pivotal role in understanding the energetics of chemical transformations. Imagine yourself as a master chef, meticulously measuring the ingredients and monitoring the temperature changes as you craft a culinary masterpiece. The heat of reaction is your secret ingredient, revealing insights into the inner workings of chemical reactions.
One of the key concepts that governs heat of reaction is bond energy. It represents the strength of the chemical bonds that hold atoms together within a molecule. Just like the strength of a magnet holding two iron objects, bond energy determines how much energy it takes to break or form those bonds.
Now, let’s visualize a chemical reaction as a rearrangement of atoms, breaking old bonds and forming new ones. When bonds are broken, energy is absorbed. This is because it takes energy to overcome the attractive forces holding the atoms together. Conversely, when new bonds are formed, energy is released. The stronger the newly formed bonds, the greater the amount of energy released.
The interplay between bond energies and heat of reaction can be beautifully illustrated through exothermic and endothermic reactions. In an exothermic reaction, the energy released by the formation of new bonds exceeds the energy absorbed by breaking the old bonds. This excess energy is typically released in the form of heat, raising the temperature of the surroundings. On the other hand, in an endothermic reaction, the energy absorbed by breaking the old bonds outstrips the energy released by forming the new bonds. This deficiency in energy must be drawn from the environment, causing a decrease in the temperature.
By understanding the concept of bond energy, chemists can unravel the intricate dance of energy exchange in chemical reactions. This knowledge not only enhances our comprehension of fundamental chemical principles but also empowers us to design and optimize reactions for specific purposes, unlocking the potential for advancements in various fields, from medicine to materials science.
Unveiling the Secrets of Chemical Heat Changes
The heat of reaction is a crucial concept in chemistry, unveiling the enthalpy change associated with chemical reactions. Enthalpy represents the total energy of a system, including its internal energy and pressure-volume work. Understanding the heat of reaction allows us to predict the energy released or absorbed during chemical processes.
Hess’s Law: A Key to Enthalpy Calculations
Hess’s law provides a valuable tool for calculating enthalpy changes in reactions. It states that the overall enthalpy change for a reaction is equal to the sum of the enthalpy changes for individual steps that lead to the final products. Hess’s law simplifies complex reactions, enabling us to break them down into simpler components.
Standard Enthalpy of Formation: A Reference Point
The standard enthalpy of formation (ΔHf°) is the enthalpy change associated with the formation of one mole of a compound from its elemental constituents under standard conditions. It serves as a reference point for enthalpy calculations, allowing us to predict the enthalpy change for any given reaction.
Thermochemical Equations: Translating Reactions into Numbers
Thermochemical equations represent chemical reactions with their corresponding enthalpy changes. These equations provide a convenient way to calculate the heat of reaction by summing up the enthalpy changes for each individual species in the reaction.
Calorimetry: Measuring Heat Changes Directly
Calorimetry is a technique that allows us to measure heat changes in reactions directly. A calorimeter, an insulated container, records the temperature change associated with a reaction. By knowing the heat capacity of the calorimeter and its contents, we can accurately determine the heat released or absorbed during the reaction.
Bond Energy and Heat of Reaction
Bond energy represents the energy required to break a bond between two atoms. Understanding bond energies helps us predict the heat of reaction, as bond breaking during reactions requires energy input, while bond formation releases energy.
Heat Capacity: Predicting Temperature Changes
Heat capacity measures the ability of a substance to absorb or release heat without significantly changing its temperature. A substance with a high heat capacity will undergo smaller temperature changes than a substance with a low heat capacity when equal amounts of heat are added or removed. Understanding heat capacity helps us predict temperature changes during reactions.
Calculating the Heat of Reaction: A Guide to Enthalpy and Hess’s Law
In chemistry, understanding the heat involved in reactions is crucial. Calculating the heat of reaction helps us predict the energy changes that occur and determine the feasibility of reactions. The enthalpy of reaction, symbolized by ΔH, measures this energy change.
Hess’s Law
Hess’s law, named after the Swiss chemist Germain Hess, simplifies the calculation of enthalpy changes for complex reactions. It states that the enthalpy change for a reaction is the sum of the enthalpy changes for the individual steps involved in the reaction. This powerful tool allows us to calculate enthalpy changes for reactions that may be difficult to measure directly.
Standard Enthalpy of Formation
The standard enthalpy of formation (ΔHf°) of a substance represents the enthalpy change when one mole of the substance is formed from its constituent elements in their standard states. This value is a useful reference point for calculating enthalpy changes in reactions.
Thermochemical Equations
Thermochemical equations are balanced chemical equations that include enthalpy changes as part of the equation. They provide a convenient way to represent the energy changes associated with reactions. By analyzing these equations, we can determine the heat of reaction for specific reactions.
Bond Energy and Calorimetry
Bond energy refers to the energy required to break a chemical bond. Knowledge of bond energies allows us to estimate the enthalpy change in reactions and predict the stability of molecules. Calorimetry is a technique used to measure heat changes in chemical reactions. This method involves measuring the temperature change in a known amount of substance or solution during a reaction.
Heat Capacity
Heat capacity (C) quantifies the amount of heat energy required to raise the temperature of a substance by one degree Celsius (or Kelvin). It is a substance-specific property that determines how much heat is absorbed or released during temperature changes.
In processes involving heat transfer, heat capacity is a critical factor. By understanding its role, we can predict temperature changes in reactions, design efficient cooling or heating systems, and optimize energy transfer processes.
Calculating the Heat of Reaction: A Comprehensive Guide
In the realm of chemistry, understanding the heat of reaction is paramount for unraveling the energetic transformations that drive chemical processes. Heat of reaction measures the energy released or absorbed during a chemical transformation, and it plays a crucial role in predicting the feasibility and outcomes of reactions.
Hess’s Law: A Bridge between Reactions
Hess’s law, a fundamental principle in thermochemistry, provides a powerful tool for calculating enthalpy changes in reactions. It states that the enthalpy change for a reaction is independent of the pathway by which the reactants are converted into products. This allows us to calculate enthalpy changes for complex reactions by breaking them down into simpler, known steps.
Standard Enthalpy of Formation: A Reference for Reaction Energies
The standard enthalpy of formation (ΔHf°) is a measure of the enthalpy change associated with the formation of one mole of a compound from its constituent elements in their standard states. By knowing the standard enthalpy of formation of reactants and products, we can calculate the enthalpy change for a reaction using Hess’s law.
Thermochemical Equations: A Mathematical Description of Heat Changes
Thermochemical equations provide a concise representation of chemical reactions, including the enthalpy change that accompanies them. The enthalpy change is expressed as a term on the right-hand side of the equation, with either a positive or negative sign indicating whether heat is released or absorbed, respectively.
Bond Energy and Calorimetry: Unveiling Energy Changes at the Molecular Level
Bond energy refers to the energy required to break a chemical bond, while calorimetry is a technique used to measure heat changes in reactions. Understanding bond energies allows us to estimate the enthalpy change for reactions involving bond breaking and formation. Calorimetry provides experimental data on the heat released or absorbed during chemical reactions.
Heat Capacity: Predicting Temperature Changes in Reactions
Heat capacity is a physical property that measures the amount of heat required to raise the temperature of one gram of a substance by one degree Celsius. Heat capacity helps us predict temperature changes in reactions. Reactions with a high heat capacity require more heat to produce a given temperature change, while reactions with a low heat capacity undergo larger temperature changes for the same amount of heat input.
By understanding the concept of heat capacity, we can accurately predict the temperature changes that occur in chemical reactions. This knowledge is essential for controlling reaction temperatures and optimizing reaction conditions in various chemical processes.