Understanding Fault Formation: Types Of Stress And Their Impact
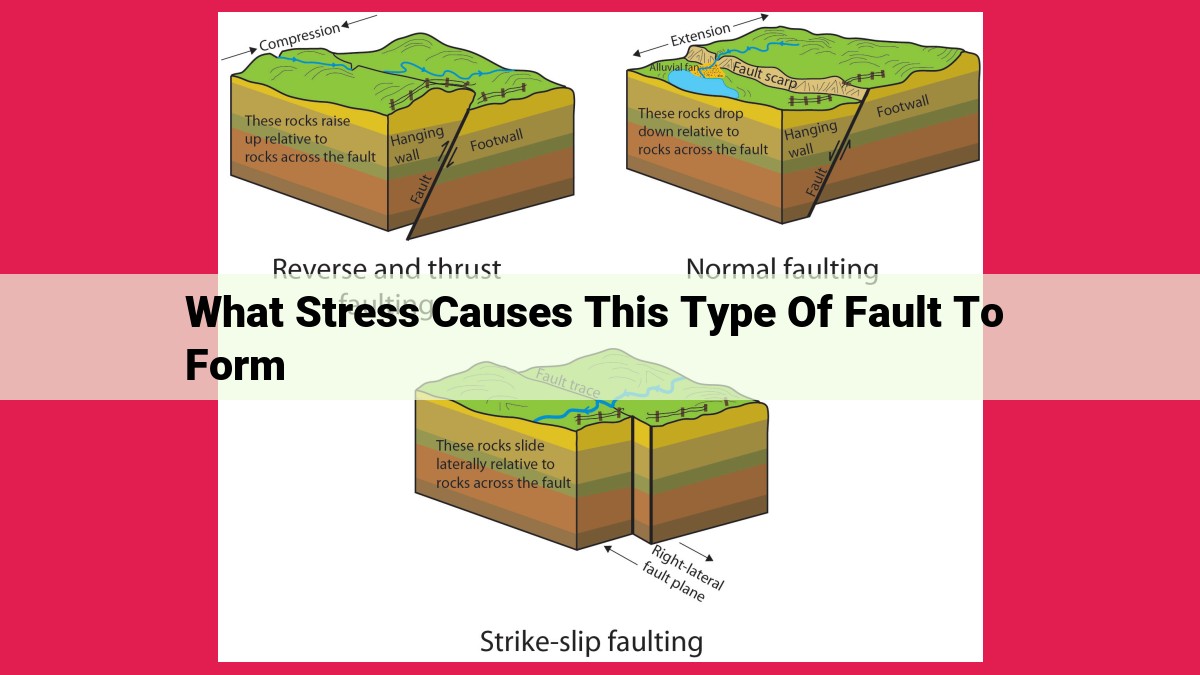
Normal stress, a force acting perpendicular to a surface, creates tension or compression. Tensional stress pulls the surface apart, causing normal faults. Shear stress, a force acting parallel to a surface, can produce strike-slip faults when surfaces move horizontally past each other. Lastly, compressive stress, a force pushing surfaces together, leads to thrust faults where one surface moves above another.
Understanding Stress Types: The Hidden Forces Shaping Earth’s Faults
When it comes to the intricate tapestry of Earth’s crust, faults stand as silent witnesses to the relentless forces that have shaped our planet over eons. At their core, these fractures in the Earth’s surface are the result of stress, a force that pushes or pulls on rocks, causing them to break and shift.
Stress comes in various forms, each leaving its unique mark on the rocks it encounters. Normal stress acts perpendicular to the rock surface, comparable to the force you apply when pushing a book on a table. Shear stress, on the other hand, occurs when two forces act parallel to the surface, causing the rock to slide past itself, akin to moving a book across the table’s surface.
Compressive stress squeezes a rock from both sides, forcing it to shorten, while tensile stress stretches it, causing it to elongate. Each of these stress types plays a pivotal role in the formation and characteristics of faults, leaving behind a story etched in the Earth’s crust.
Fault Plane Orientation: Understanding the Anatomy of Faults
When the Earth’s crust succumbs to the relentless forces of stress, it often responds by fracturing along planes of weakness, creating faults. These faults are not mere cracks but complex structures with distinct orientations that reveal the forces that shaped them.
Strike, Dip, and Rake: The Three Musketeers of Fault Orientation
Just as a compass guides a traveler through the wilderness, the concepts of strike, dip, and rake serve as the cartographers of fault planes. Strike measures the fault’s horizontal compass direction, while dip quantifies the angle at which it plunges beneath the Earth’s surface. Finally, rake indicates the direction of motion along the fault, providing crucial insights into the stress regime that created it.
Strike: Imagine a fault as a giant wall slicing through the Earth’s crust. The strike is simply the direction you would face if you were standing perpendicular to this wall. Just like the North Star guides navigators, strike provides a reference point for understanding the overall orientation of the fault.
Dip: The dip, on the other hand, tells you how steeply the fault dips into the subsurface. Think of it as the angle you would measure if you were standing on one side of the fault and looking across to the other. High-angle faults dip steeply, while low-angle faults lie more gently beneath the Earth’s surface.
Rake: Lastly, rake describes the direction in which the rocks on one side of the fault have moved relative to the rocks on the other side. It’s like a dance between two tectonic plates, where one slides past the other in a specific direction. Rake provides vital clues about the type of fault and the stress conditions that caused it to form.
Influence of Rock Type on Fault Formation
In the realm of geology, the quest to unravel the secrets of fault formation is a captivating endeavor. Among the key factors that determine the type of fault that arises is rock type. Different rocks possess distinct characteristics that govern their susceptibility to deformation and fracture.
Brittle Rocks: A Stubborn Resistance
Brittle rocks, like the unyielding granite, exhibit minimal deformation under stress. When these rocks reach their breaking point, they shatter abruptly, forming clean breaks known as faults. The resulting faults are commonly characterized by steep dips and sharp edges, reflecting the brittle nature of the rock.
Ductile Rocks: A Graceful Surrender
In contrast, ductile rocks such as malleable sandstone can withstand significant deformation without rupturing. These rocks undergo a gradual process of bending and folding under stress, allowing them to accommodate strain energy without catastrophic failure. Faults formed in ductile rocks tend to have gentler dips and more complex geometries, reflecting the ductile behavior of the rock.
The contrasting behaviors of brittle and ductile rocks stem from their internal structures. Brittle rocks possess strong interatomic bonds that resist deformation, while ductile rocks have weaker bonds that allow for significant atomic rearrangement.
Understanding the role of rock type in fault formation is crucial for geologists seeking to unravel the tectonic history of a region. The presence of certain fault types can provide valuable clues about the types of stress regimes and rock sequences that shaped the area’s geological past.
Temperature Considerations
Temperature plays a crucial role in fault formation, offering insights into the interplay between stress and rock properties. Two critical temperatures that influence fault behavior are the melting temperature and the Curie temperature.
Melting Temperature
The melting temperature is the point at which a rock transitions from a solid to a liquid state. When rocks are subjected to extreme stress and elevated temperatures, they can reach their melting temperature. This leads to the formation of magma, which weakens the rock and reduces its ability to withstand stress. As a result, faults are more likely to form in rocks that approach or reach their melting temperatures.
Curie Temperature
The Curie temperature is the temperature at which certain magnetic minerals lose their magnetic properties. This loss of magnetism is caused by the breakdown of the mineral’s atomic structure due to increased temperature. In the context of fault formation, the Curie temperature is significant because it can provide information about the temperature and stress conditions under which a fault formed. By studying the magnetic properties of rocks near faults, geologists can infer the temperature and stress conditions that existed during fault formation.
In summary, understanding the significance of melting temperature and Curie temperature helps geologists unravel the complex interplay between temperature, stress, and rock properties in the formation of faults.
Pressure and Fault Formation: Unveiling the Role of Pressure in Earth’s Dynamics
In the intricate dance of geological forces, pressure plays a pivotal role in shaping the Earth’s crust. Its influence on fault formation is a captivating tale that reveals the complex interplay of stress, rock type, and temperature.
Lithostatic pressure, the weight of the overlying rock layers, exerts a tremendous force that can deform and fracture rock. Hydrostatic pressure, the pressure exerted by fluids such as water or magma within pores and fractures, can counterbalance lithostatic pressure, reducing the likelihood of fault formation.
Confinement pressure, the pressure acting perpendicular to the direction of stress, is crucial in determining fault type. High confining pressure tends to promote ductile deformation, leading to the formation of faults with less visible displacement.
The Interplay of Pressure and Stress
Under the right conditions, the interaction of pressure and stress can trigger fault formation. When stress exceeds the strength of the rock, it yields, creating a fracture. The type of fault formed depends on the orientation of the stress relative to the rock layers (stress field).
Influence of Rock Type
Rock type plays a significant role in mediating the effects of pressure and stress on fault formation. Brittle rocks, such as granite, are more prone to fracturing under high stress and low confining pressure. Ductile rocks, such as sandstone, tend to deform plastically, resulting in faults with gradual displacement over time.
Pressure is a hidden force that profoundly influences fault formation, shaping the Earth’s crust and contributing to the dynamic processes that craft our planet. Understanding the interplay of pressure, stress, rock type, and temperature provides a window into the intricate workings of our geological world.