Understanding Emf (Electromotive Force): Key Concepts, Applications, And Optimization
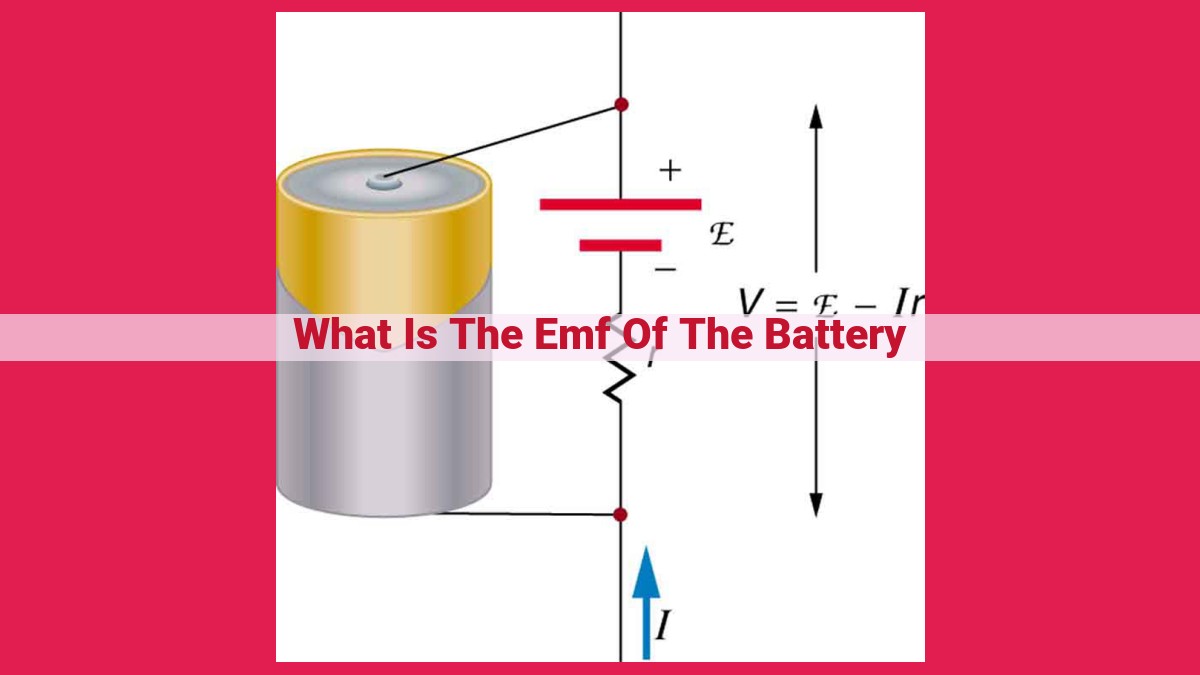
EMF (electromotive force) is the electrical potential difference produced by a battery, representing the potential difference between its terminals. Measured in volts, EMF arises from electrochemical reactions within the battery, converting chemical energy into electrical energy. It provides the power to devices and circuits, flowing through a closed circuit and generating current. EMF is also important for understanding battery efficiency and comparing power output.
- Define EMF as the electrical potential difference produced by a battery or electrochemical cell.
Electromotive Force (EMF): The Spark of Electrical Potential
In the realm of electricity, electromotive force (EMF) stands as the driving force behind the flow of electrons. It’s the electrical potential difference that fuels batteries and electrochemical cells, enabling them to convert chemical energy into the electricity powering our devices.
EMF is measured in volts, representing the electrical potential or voltage difference between the terminals of a battery. This voltage is generated by electric fields and forces within the electrochemical cell, creating a path for electrons to move.
Batteries and EMF: The Powerhouses of Electrical Energy
Batteries are essentially electrochemical cells that harness chemical energy to produce electrical energy. Inside a battery, chemical reactions between different materials generate an EMF. This EMF creates a potential difference between the battery’s positive and negative terminals, allowing current to flow when connected to a circuit.
EMF: A Measure of Electrical Potential
Understanding the concept of electromotive force (EMF) is essential for comprehending the fundamentals of batteries, electrical circuits, and the flow of electrical energy. EMF, often referred to as voltage, plays a pivotal role in powering our electronic devices and enabling various electrical applications.
Measuring EMF: A Story of Potential Difference
In the realm of electricity, the potential difference between two points, often measured in volts, provides a measure of the electrical potential or the ability to do electrical work. Just as water flows from a higher elevation to a lower one, electrical charge tends to move from areas of higher electrical potential to areas of lower electrical potential. This flow of charge constitutes an electric current.
The Role of Electric Fields in Generating EMF
To understand the origin of EMF, we delve into the world of electric fields. These fields are regions of space where electric charges exert forces on each other. When a battery is connected to a circuit, it creates an electric field that pushes positive charges in one direction and negative charges in the opposite direction. This separation of charges creates a potential difference across the battery, giving rise to EMF.
Batteries and EMF: The Heart of Electrical Power
Batteries are electrochemical cells that serve as a source of EMF. They consist of two electrodes immersed in an electrolyte solution. Chemical reactions within batteries generate an electrical potential difference between the electrodes, resulting in the flow of electrons and the production of an electric current. This process is the foundation of battery-powered devices.
Batteries and EMF: The Powerhouse of Electrical Energy
Batteries are the unsung heroes of our modern world, powering everything from our smartphones to electric cars. But how do these remarkable devices generate the electrical energy that drives our devices? The answer lies in a fundamental concept known as Electromotive Force (EMF).
EMF: The Heart of Battery Power
EMF is the electrical potential difference produced by a battery. It’s like a voltage pump that creates the driving force for electrons to flow through a circuit. Batteries are essentially electrochemical cells that convert chemical energy stored in their internal structure into electrical energy.
Electrochemical Reactions: The Spark of Electricity
Inside a battery, electrochemical reactions take place between different chemicals, such as zinc and manganese dioxide. These reactions release electrons, creating an imbalance of charge within the battery. This charge imbalance generates electric charge, which is the driving force behind EMF.
Terminal Voltage: The Measured Power
The terminal voltage of a battery is the voltage measured across its terminals. It’s not always equal to its EMF due to internal resistance within the battery. Internal resistance is like a small resistor that restricts the flow of electrons, causing a voltage drop.
Understanding Battery Efficiency
The efficiency of a battery depends on its internal resistance. A battery with low internal resistance will have a higher terminal voltage and deliver more power. However, all batteries have some degree of internal resistance, which is why they can’t deliver their full EMF in all situations.
Practical Applications: Powering Our Devices
EMF is the driving force behind the electrical energy that powers our devices. From the batteries in our laptops to the generators in our power plants, EMF plays a crucial role in delivering the current that keeps our world running.
Chemical Energy and EMF: Fueling Electrical Power
In the realm of electricity, energy is not simply a constant; it transforms from one form to another. Chemical energy, a hidden power source within the bonds of molecules, holds the key to unlocking electromotive force (EMF), the driving force behind electrical devices.
Chemical reactions, like microscopic fireworks, release energy when chemical bonds break and form anew. This energy manifests as heat, but in a battery or electrochemical cell, it’s harnessed differently. Within these energy-storing capsules, chemical bonds fuel a dance of charged particles, generating an electrical potential.
Fuel, the raw material for this chemical transformation, is consumed as bonds break and release their stored energy. Heat, a byproduct of many reactions, can also play a role in the efficiency of the process. Understanding the intricate connection between chemical energy and EMF is crucial for comprehending the heart of batteries and electrochemical cells.
Electrical Energy and EMF: Powering Devices
In the realm of electricity, electromotive force (EMF) reigns supreme, providing the impetus for electrons to flow, illuminating our homes, and powering the myriad of devices that shape our modern lives. EMF, the electrical potential difference between two points, acts as the driving force, enabling the conversion of chemical energy stored in batteries into the electrical energy that powers our world.
The Circuit: A Path for Electrical Energy
Just as water flows downhill, electrical energy seeks the path of least resistance, following a circuit that connects the terminals of an energy source, such as a battery. Within this circuit, electrical devices act as consumers, tapping into the electrical energy to perform their designated functions.
EMF: The Driving Force
EMF, measured in volts, represents the potential difference between the terminals of an energy source. This potential difference, akin to a pressure gradient, drives the flow of electrons through the circuit, initiating the transfer of electrical energy.
Resistance: Controlling the Flow
As electrons embark on their journey through the circuit, they encounter resistance, a property of materials that impedes the flow of current. Resistance, measured in ohms, acts as a gatekeeper, regulating the amount of current that can pass through a given path.
Voltage, Current, and Power: The Dynamic Trio
The interplay between EMF, resistance, and current forms the foundation of electrical circuits. EMF propels the electrons, resistance governs their flow, and current quantifies the number of electrons passing through a point in time. Together, these factors determine the power dissipated by the circuit, measured in watts, a measure of the rate at which electrical energy is transferred.
Example: The Light Bulb’s Glow
Consider a light bulb connected to a battery. The EMF of the battery provides the potential difference that drives the electrons through the bulb’s filament. Resistance within the filament limits the current flow, ensuring that the filament does not overheat and burn out. As electrons traverse the filament, their energy is converted into light and heat, illuminating the bulb and adding warmth to the room.
Open Circuits and EMF: Measuring True Potential
In the realm of electricity, electromotive force (EMF) plays a crucial role in determining the electrical potential within a circuit. An open circuit presents a unique scenario where this potential can be directly measured.
In an open circuit, the pathway for current flow is interrupted, resulting in zero current (I). Consequently, the resistance (R) in the circuit becomes infinite. This infinite resistance creates an intriguing situation where the EMF of the circuit is directly manifested as a potential difference (V) across the open circuit.
In such a scenario, it’s as if the electrical energy is eager to flow, but the lack of a complete circuit prevents its movement. The EMF represents the full electrical potential waiting to be unleashed.
Measuring the EMF in an open circuit is like taking a snapshot of the circuit’s inherent potential, unaffected by any external influences. It provides a true and direct measure of the electrical force that the circuit is capable of generating.
By understanding the significance of open circuits and EMF, we gain a deeper appreciation for the intricate interplay of electrical forces within circuits. It allows us to accurately assess the potential of electrical systems and optimize their performance for various applications.
Closed Circuits and EMF: Generating Current
In the realm of electricity, the flow of current holds the key to powering our devices and illuminating our lives. In a closed circuit, where a complete loop allows electrons to embark on an uninterrupted journey, Electromotive Force (EMF) plays the pivotal role of setting electrons in motion.
When a closed circuit is established, the EMF acts as the driving force, propelling electrons through the wires, like a tireless shepherd guiding its flock. As the electrons navigate the circuit, they encounter resistance, akin to obstacles in their path. This resistance impedes their progress, resulting in a voltage drop along the circuit.
The relationship between EMF, current, resistance, and voltage drop can be elegantly captured by Ohm’s Law:
EMF = Current × Resistance + Voltage Drop
This equation reveals that the EMF is the sum of the voltage drop across the resistance and the voltage needed to drive the current. In essence, EMF provides the energy to overcome resistance and sustain the flow of current.
Example: Consider a closed circuit with an EMF of 6 volts, a resistance of 2 ohms, and a current of 3 amperes. Using Ohm’s Law, we can calculate the voltage drop across the resistance:
Voltage drop = Current × Resistance = 3 A × 2 Ω = 6 V
The calculated voltage drop of 6 volts is equal to the EMF, indicating that all the energy provided by the EMF is used to drive current through the resistance.
Internal Resistance and EMF: Understanding Battery Efficiency
When exploring the fascinating world of batteries and electrochemical cells, one crucial concept that demands our attention is internal resistance. Internal resistance refers to the resistance inherent within a battery, a characteristic that significantly influences its efficiency and energy output.
As we delve into the realm of batteries, we uncover their intricate inner workings. These electrochemical powerhouses generate electricity through chemical reactions, creating a potential difference between their terminals. This potential difference, known as electromotive force (EMF), represents the battery’s capacity to drive current flow.
However, the flow of electrons through a battery is not without hindrance. Within the battery’s internal structure, we encounter a resistive force that opposes this electron movement: internal resistance. This resistance arises from various factors, including the battery’s construction materials, electrode design, and electrolyte composition.
Internal resistance plays a critical role in determining a battery’s efficiency, which measures its ability to convert stored chemical energy into electrical energy. A battery with higher internal resistance will experience greater energy loss as heat, reducing its overall efficiency. This energy loss manifests as a voltage drop across the battery’s terminals when it is connected to a load.
To fully grasp the impact of internal resistance, we turn to Ohm’s law, a fundamental principle in the realm of electricity. Ohm’s law establishes a direct relationship between voltage, current, and resistance. In the context of batteries, Ohm’s law reveals that the terminal voltage (V), or the voltage measured across the battery’s terminals, is directly proportional to the EMF and inversely proportional to the sum of the internal resistance (r) and the external load resistance (R):
V = EMF - (r + R) * I
Here, I represents the current flowing through the circuit. This equation illuminates the interplay between EMF, internal resistance, and circuit load in determining the terminal voltage. A higher EMF or lower internal resistance will result in a higher terminal voltage, ensuring more efficient power delivery.
Understanding internal resistance is paramount in selecting batteries for specific applications. Batteries with lower internal resistance are better suited for high-power applications, where they can deliver substantial current without excessive energy loss. Conversely, batteries with higher internal resistance are more appropriate for low-power applications, where efficiency is less critical.
By considering internal resistance alongside EMF, we gain a deeper understanding of battery performance and can optimize our choices for various electronic devices and systems. Whether powering portable electronics, electric vehicles, or industrial machinery, batteries remain indispensable energy sources, and their internal resistance characteristics play a pivotal role in their effectiveness.
Terminal Voltage and EMF: Comparing Power Output
In the realm of batteries and electrical circuitry, the concepts of Electromotive Force (EMF) and Terminal Voltage play crucial roles in understanding the performance and efficiency of these systems. While EMF represents the internal potential difference of a battery, terminal voltage measures the voltage actually output by the battery under various operating conditions.
To comprehend this relationship, it’s essential to grasp the concept of internal resistance. Every battery possesses a certain amount of internal resistance due to various factors like chemical composition and physical design. This internal resistance acts as a resistor within the battery, opposing the flow of current.
As current flows through a closed circuit, the terminal voltage drops below the EMF of the battery. This voltage drop is directly proportional to the current and the internal resistance of the battery. In other words, as the current increases, the voltage drop across the internal resistance increases, resulting in a lower terminal voltage.
The relationship between EMF, internal resistance, and circuit load can be encapsulated by the following equation:
Terminal Voltage = EMF - (Internal Resistance * Current)
This equation reveals that the terminal voltage is influenced by both the EMF of the battery and the internal resistance. The higher the internal resistance of a battery, the greater the voltage drop for a given current. This means that a battery with high internal resistance will deliver a lower terminal voltage compared to a battery with low internal resistance.
Understanding this relationship is critical when selecting and using batteries for various applications. Batteries with low internal resistance are preferred for high-current applications, as they can maintain a higher terminal voltage under load. Conversely, batteries with high internal resistance are more suitable for low-current applications, where voltage regulation is less critical.
In conclusion, terminal voltage provides valuable insights into the performance and efficiency of batteries. By considering the interplay between EMF, internal resistance, and circuit load, we can optimize the selection and usage of batteries to meet the specific requirements of different electrical devices and systems.