Understanding Electron Configuration: Arrangement Of Electrons In An Atom
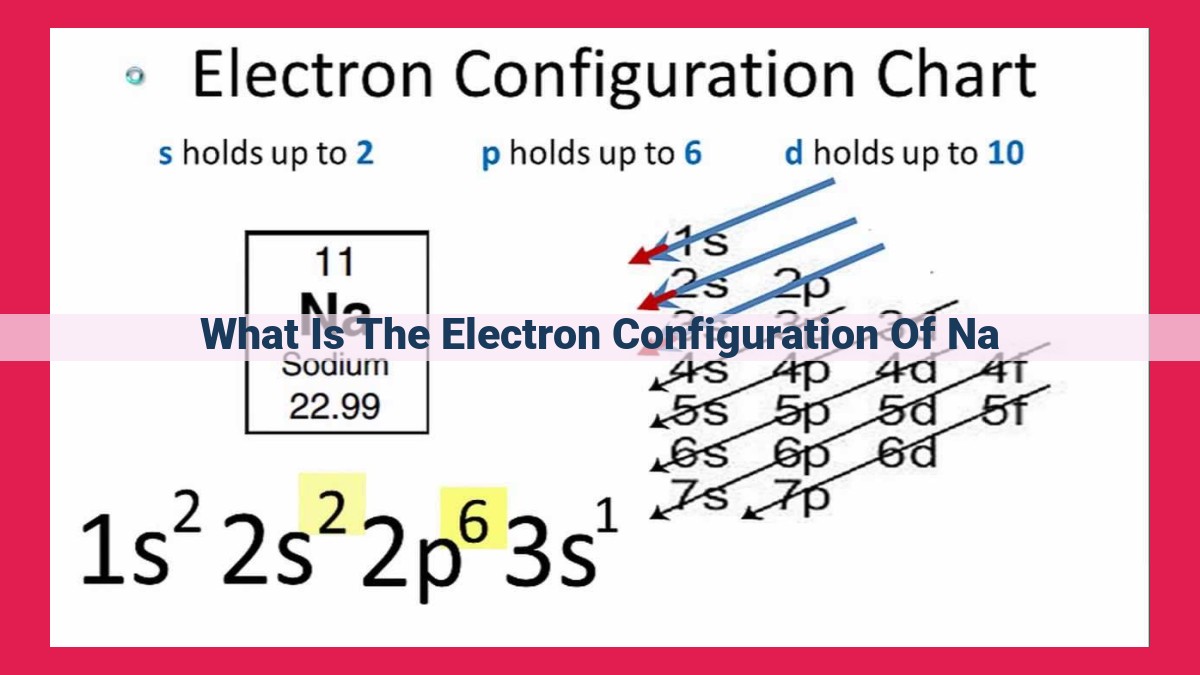
The electron configuration of an atom describes the arrangement of electrons around its nucleus. It is determined based on the atomic number, which directly corresponds to the number of electrons. Using the Aufbau principle, electrons fill atomic orbitals sequentially from lowest to highest energy levels and specific sublevel sequences. The electron configuration is represented using numbers and letters. The electron configuration of sodium (Na) is an illustrative example, with its 11 electrons arranged as 1s²2s²2p⁶3s¹. This configuration provides insights into atomic properties such as size, ionization energy, and chemical behavior, as electron configuration plays a crucial role in determining how elements interact and bond with each other.
Unveiling the Secrets of Electron Configuration: A Journey into the Heart of Matter
Imagine yourself as an explorer embarking on a quest to uncover the hidden secrets of an atom’s interior. Your mission? To unravel the intricate arrangement of electrons that dance around an atom’s nucleus, a mystery known as electron configuration. This knowledge holds the key to understanding the very nature of matter and unlocking the marvels of chemistry.
Electron configuration is the blueprint that reveals how electrons are distributed within an atom, forming the foundation of atomic structure. It plays a pivotal role in determining an element’s chemical behavior, shaping its reactivity and defining its position on the periodic table. By deciphering the electron configuration of an atom, we can predict its atomic size, ionization energy, and electronegativity.
Understanding electron configuration is paramount in the realm of chemistry. It provides profound insights into how elements interact and form bonds, driving the intricate tapestry of chemical reactions that shape the world around us.
Atomic Number and Electron Count: Balancing the Proton Pull
In the vast expanse of the atomic realm, every element possesses a distinct identity, a signature that sets it apart from all others. This identity stems from the number of protons nestled within the atom’s core, a value known as the atomic number. Like a cosmic census, the atomic number defines an element’s place in the periodic table, revealing its unique character.
But atoms are not mere collections of protons; they also harbor a cloud of electrons, negatively charged particles that dance around the nucleus like celestial bodies. The number of electrons in an atom is not a random occurrence; it is inextricably linked to the atomic number. Protons, with their positive charge, exert a pull on electrons, drawing them into a harmonious balance. For every proton in the nucleus, there must be an equal number of electrons orbiting the atom. This delicate equilibrium ensures that the atom remains electrically neutral, without any excess or deficit of charge.
The relationship between atomic number and electron count is not just a matter of balancing charges; it also provides a glimpse into the atom’s chemical behavior. Elements with similar atomic numbers tend to share similar chemical properties, as the number of electrons in their outermost energy level determines their reactivity. Understanding this interplay between atomic number and electron count is a crucial step in unraveling the intricate tapestry of atomic structure and chemical bonding.
Aufbau Principle: The Guiding Force of Electron Filling
Imagine an atomic world where electrons dance around the nucleus like celestial bodies. The Aufbau Principle, like a cosmic choreographer, governs the order in which these electrons occupy their energy levels within the atom’s orbitals.
According to the principle, electrons fill atomic orbitals from the lowest energy levels to the highest. This means that they start by filling the orbitals closest to the nucleus, which have the lowest energy. As the orbitals become further away from the nucleus, their energy increases, and electrons will only fill them after the lower energy orbitals are occupied.
Within each energy level, there are sublevels with slightly different energies. The filling pattern follows a specific sequence: s, p, d, and f. The s sublevel has one orbital, the p sublevel has three orbitals, the d sublevel has five orbitals, and the f sublevel has seven orbitals.
Electrons will first fill the s sublevel, then the p sublevel, then the d sublevel, and finally the f sublevel. For example, the first two electrons in an atom will fill the 1s sublevel, the next six electrons will fill the 2s and 2p sublevels, and so on.
This orderly filling pattern ensures that electrons are placed in the most stable and energetically favorable configurations. It also has a profound impact on the chemical properties of elements, as the electron configuration determines an element’s size, ionization energy, and electronegativity.
Electron Configuration Notation: Depicting the Dance of Electrons
Delving into the fascinating world of chemistry, understanding how electrons dance around the nucleus of atoms is crucial. To represent this intricate arrangement, chemists employ a unique form of notation known as electron configuration. But what is this enigmatic notation all about?
Electro configuration notation is a symbolic representation that depicts the distribution of electrons within the atomic orbitals. This distribution is critical as it governs the chemical behavior of elements. The notation uses a series of numbers and letters to represent the specific orbitals and the number of electrons in each.
For instance, the notation for sodium (Na), an alkali metal with one valence electron, would be 1s²2s²2p⁶3s¹. This notation conveys that sodium has two electrons in the first energy level (1s), two in the second (2s), and six in the third energy level (2p), with one additional electron in the outermost 3s orbital.
A simplified version of electron configuration notation, known as noble gas configuration, is often used to represent common electron distributions. For example, the electron configuration of neon (Ne), a noble gas, can be written as [He]2s²2p⁶. This notation indicates that neon has the same electron configuration as helium (He) but with the addition of two electrons in the 2s orbital and six electrons in the 2p orbital.
By unraveling the intricacies of electron configuration notation, scientists gain a deeper understanding of the structure and properties of atoms. This knowledge forms the foundation of chemistry, enabling us to predict how elements will behave and interact, laying the groundwork for the development of new materials and innovations that shape our world.
Pauli Exclusion Principle: Limiting Electron Occupancy
- Statement: No two electrons can occupy the same quantum state.
- Enforcing unique electron states: Ensures specific arrangements and prevents identical electron configurations.
The Pauli Exclusion Principle: Unlocking the Quantum Dance of Electrons
Our journey into the enigmatic world of electrons brings us to a captivating principle that governs their behavior, shaping the very fabric of atoms – the Pauli Exclusion Principle. This fundamental law asserts that no two electrons can coexist in the same quantum state, a concept that holds profound implications for our understanding of atomic structure.
Imagine a stage where electrons are the performers, each with a unique set of characteristics. The quantum state of an electron encompasses its energy, shape, and spin. According to the Pauli Exclusion Principle, no two electrons can share the same stage, they cannot occupy the same quantum state. This principle ensures that each electron has a distinct identity, preventing them from becoming indistinguishable.
The Pauli Exclusion Principle acts as a choreographer, regulating the electron waltz. It dictates the specific patterns in which electrons arrange themselves within an atom. This arrangement determines the atom’s stability and governs its interactions with other atoms, ultimately shaping the properties of matter.
The Pauli Exclusion Principle not only ensures the uniqueness of electron states but also explains why certain electron configurations are forbidden. It prevents electrons from piling up in the lowest energy state, forcing them to explore higher energy levels. This phenomenon is responsible for the periodic trends observed in the properties of elements.
In the realm of chemistry, the Pauli Exclusion Principle plays a pivotal role. It determines the number of electrons that can occupy each orbital, laying the foundation for understanding chemical bonding. By comprehending the electron configurations of atoms, scientists can predict the reactivity and behavior of chemical species.
The Pauli Exclusion Principle is a cornerstone of quantum mechanics, providing a window into the enigmatic world of electrons. It reveals the intricate dance of these subatomic particles, shaping the very foundations of our universe.
Hund’s Rule: The Battle of the Spinning Electrons
In the realm of atoms, electrons dance around the nucleus, each with its own unique spin. Like tiny magnets, these spinning electrons can either align with or oppose each other. Hund’s Rule steps into the ring and declares that electrons prefer to occupy orbitals with parallel spins, maximizing their spin power.
The Parallel Preference
Electrons, like social butterflies, tend to favor the company of their “spin twins.” When faced with an empty orbital, they’ll happily occupy it with a “spin-up” sibling. If that orbital is already hosting a “spin-down” electron, the second electron will squeeze in next to it, keeping the spins parallel.
Unleashing Magnetic Mayhem
This parallel spin alignment has a profound impact on an atom’s magnetic properties. The more unpaired electrons with parallel spins an atom possesses, the stronger its magnetic susceptibility. This means that atoms with Hund’s Rule configurations are more easily influenced by magnetic fields.
The Case of Oxygen
Let’s take oxygen as an example. Its electron configuration, 1s² 2s² 2p⁴, tells us that it has two unpaired electrons in the 2p subshell. These two parallel spins make oxygen paramagnetic, meaning it’s attracted to magnetic fields.
The Importance of Hund’s Rule
Hund’s Rule is a critical player in understanding chemical bonding, reactivity, and magnetic properties of atoms. It governs electron configurations, determining the arrangement of electrons in orbitals and influencing the overall behavior of atoms in chemical interactions. By comprehending this rule, chemists can delve deeper into the secrets of atomic structure and unravel the intricacies of matter itself.
Electron Configuration of Sodium: A Case Study
Sodium, an alkali metal with the atomic number 11, serves as an excellent example for illustrating the principles of electron configuration. Let’s embark on a step-by-step determination of its electron arrangement.
1. Atomic Number and Electron Count
Sodium, with 11 protons in its nucleus, has 11 electrons to balance its positive charge. This sets the stage for constructing its electron configuration.
2. Aufbau Principle and Orbital Filling
The Aufbau Principle guides the sequential filling of orbitals, starting with the lowest energy levels. Sodium’s electrons will occupy the 1s, 2s, 2p orbitals in that order.
3. Pauli Exclusion Principle and Hund’s Rule
The Pauli Exclusion Principle dictates that no two electrons can share the same quantum state. This means each orbital can hold a maximum of two electrons. Hund’s Rule favors orbitals with parallel electron spins, maximizing the magnetic moment of the atom.
4. Electron Configuration Notation
Combining these principles, we arrive at sodium’s electron configuration: 1s²2s²2p⁶3s¹. This notation indicates that the first energy level (1s) has two electrons, the second level (2s) has two electrons, the 2p sublevel has six electrons, and the third level (3s) has one electron.
5. Significance for Sodium’s Properties
Sodium’s electron configuration directly influences its atomic properties and chemical behavior. Its single 3s¹ electron makes sodium highly reactive and prone to form ionic bonds. This characteristic underlies its essential role in biological processes, such as maintaining fluid balance and regulating nerve function.
The electron configuration of sodium offers a glimpse into the fundamental structure of matter. By understanding the principles governing electron arrangements, scientists can predict the properties and behavior of elements, paving the way for advancements in diverse fields, including chemistry, materials science, and biology.
Predicting Atomic Properties and Chemical Behavior: The Role of Electron Configuration
As we delve into the fascinating world of electron configuration, we unveil its profound influence on the realm of atomic properties and chemical behavior.
Electron Configuration: A Blueprint for Properties
The arrangement of electrons around an atom’s nucleus, known as electron configuration, holds the key to predicting several crucial atomic properties. These properties include:
- Atomic Size: The size of an atom is dictated by the number of electron shells. More electron shells lead to a larger atom.
- Ionization Energy: The energy required to remove an electron from an atom is called ionization energy. It is affected by the number of electrons and the distance from the nucleus.
- Electronegativity: The tendency of an atom to attract electrons in a chemical bond is known as electronegativity. Electron configuration strongly influences electronegativity.
Chemical Bonding: A Dance of Electron Configurations
The chemical behavior of elements is deeply intertwined with their electron configurations. The outermost electrons, known as valence electrons, play a pivotal role in determining how elements interact.
- Ionic Bonding: When an element has a strong tendency to lose or gain electrons, it can form ionic bonds.
- Covalent Bonding: Elements with similar electronegativities tend to form covalent bonds, sharing electrons to achieve a stable configuration.
Electron configuration serves as a fundamental tool for understanding the behavior of atoms and their interactions. By deciphering the intricate arrangement of electrons, scientists can predict atomic properties and unravel the secrets of chemical bonding. This knowledge forms the cornerstone of modern chemistry and materials science, enabling breakthroughs in countless fields.