Electrochemical Gradient: Understanding The Driving Force For Ion Movement Across Cell Membranes
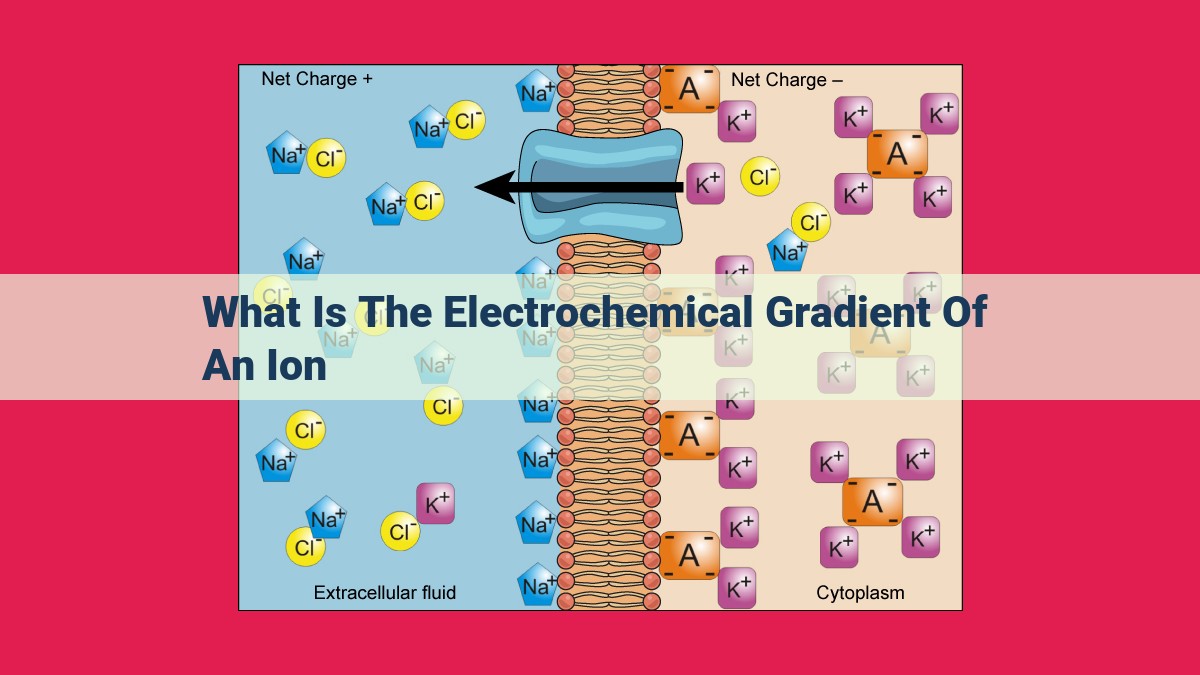
The electrochemical gradient of an ion describes the combined force driving its movement across a cell membrane. It consists of two components: an ion concentration gradient, where ions are more concentrated on one side of the membrane, and a membrane potential, an electrical difference across the membrane. The electrochemical gradient determines the direction and rate of ion movement through ion channels or transporters, playing a crucial role in maintaining cellular homeostasis, nerve impulses, and muscle function.
Understanding the Electrochemical Gradient: A Journey into Ion Transport
In the realm of biology, the electrochemical gradient emerges as a key player in the movement of ions across cellular membranes. This gradient, a combination of ion concentration and membrane potential, serves as the driving force that propels ions through these biological barriers.
The Electrochemical Gradient’s Role in Ion Transport
Imagine a river flowing down a mountain, its force fueled by the difference in height between its source and its destination. In the same way, the electrochemical gradient harnesses the concentration difference of ions across a membrane and the electrical difference between the two sides of the membrane to drive ions in a specific direction.
Positive ions (cations) like sodium (Na+) and calcium (Ca2+) are attracted to negative charges inside the cell, while negative ions (anions) like chloride (Cl-) and bicarbonate (HCO3-) are drawn to positive charges outside. This concentration gradient creates a potential difference across the membrane, known as the membrane potential.
The combination of the concentration gradient and the membrane potential gives rise to the electrochemical gradient. This gradient exerts a force on ions, pushing them towards the area of lower concentration or opposite charge, thereby facilitating their movement across the membrane.
This movement of ions is crucial for a wide range of biological processes, including nerve impulses, muscle contractions, and the regulation of cellular pH. Understanding the electrochemical gradient provides a fundamental insight into the workings of these essential biological functions.
Unveiling the Electrochemical Gradient: The Driving Force Behind Ion Movement
The Enigma of Electrochemical Gradient
In the intricate world of cells, an invisible gateway exists – the electrochemical gradient. This gradient acts as a driving force, guiding the movement of ions across the selectively permeable cell membrane. Understanding this gradient is crucial to comprehending cellular functions, especially ion transport.
Delving into the Components
The electrochemical gradient consists of two interconnected components: ion concentration gradient and membrane potential. The ion concentration gradient refers to the difference in the concentration of a specific ion on either side of the membrane. For instance, potassium ions (K+) are more concentrated inside the cell than outside, creating an ion concentration gradient.
The membrane potential, on the other hand, arises from the unequal distribution of charged ions across the membrane. This creates an electrical field, with the inside of the cell typically being negative relative to the outside. The membrane potential influences the movement of ions based on their charge; positively charged ions are attracted to the negative interior, while negatively charged ions are repelled.
Driving Ion Movement
The electrochemical gradient acts as a compass, guiding the movement of ions across the membrane. Ions tend to move down their concentration gradient, from areas of higher concentration to lower concentration. Simultaneously, they are influenced by the membrane potential, which either facilitates or hinders their movement based on their charge.
A Delicate Balance
The electrochemical gradient is a dynamic equilibrium, constantly fine-tuned by the cell to maintain its delicate balance. Selective membrane channels regulate the passage of ions, altering the ion concentration gradient and membrane potential as needed.
Significance: Acellular Symphony
The electrochemical gradient is not merely a concept but a vital force that governs a symphony of cellular processes. It drives passive ion transport, allowing ions to flow down their gradient without energy input. It also plays a crucial role in active ion transport, where energy is utilized to move ions against their gradient.
The electrochemical gradient is particularly critical in nerve and muscle function. In nerve cells, it generates the electrical signals that transmit information throughout the body. In muscle cells, it triggers the contractions that enable movement.
Comprehending the electrochemical gradient is fundamental to understanding cellular function. This invisible gateway controls the movement of ions across membranes, shaping cellular processes and the very essence of life itself. By unraveling the secrets of the electrochemical gradient, we unlock a deeper appreciation for the intricate workings of the cellular world.
Exploring Membrane Potential: The Electrical Gatekeeper
In the realm of ion transport, the membrane potential emerges as an electrical gatekeeper, orchestrating the flow of ions across cellular membranes. It manifests as an electrical difference, a voltage disparity between the membrane’s two sides. This voltage gradient exerts a profound influence on the ion flux, the net movement of ions, across the membrane.
Imagine a membrane as a selectively permeable barrier, like a semi-porous wall. Ions, charged particles, are constantly striving to move down their concentration gradient, from areas of high concentration to areas of low concentration. However, the membrane potential acts as a force that opposes this movement, either encouraging or hindering it.
Negative membrane potential arises when the inside of the membrane is negatively charged relative to the outside. This negative charge attracts positively charged ions (cations), drawing them towards the inside. Conversely, it repels negatively charged ions (anions), pushing them towards the outside.
Positive membrane potential, on the other hand, has the opposite effect. It attracts anions and repels cations. The magnitude and direction of the membrane potential determine the strength and direction of the ion flux.
Understanding membrane potential is crucial for unraveling the intricate workings of biological systems. It plays a pivotal role in transmitting signals within neurons, triggering muscle contractions, and regulating the ionic balance within cells. Its disruption can lead to a cascade of physiological abnormalities, underscoring its profound impact on cellular function.
The Ion Concentration Gradient: The Driving Force Behind Ion Movement
In the bustling world of ion transport, a force reigns supreme: the ion concentration gradient. Imagine a crowd of thirsty ions, eager to quench their thirst on the other side of a crowded membrane. This gradient, a difference in ion concentrations between two compartments, serves as the driving force that propels these ions across the membrane barrier.
Without this gradient, the ions would remain trapped, like cars stuck in traffic. But when a concentration difference exists, it’s like the traffic jam has been cleared, allowing the ions to flow freely. The higher the concentration difference, the more ions rush to balance out the disparity. This movement creates a flow of ions, an electric current that plays a crucial role in biological processes.
The ion concentration gradient is not just a passive force; it’s an active player in shaping cellular processes. It’s the spark that ignites passive ion transport, a process where ions move down their concentration gradients, from high to low. This effortless movement is essential for maintaining electrical balance and transporting ions across membranes.
Moreover, the ion concentration gradient provides the impetus for active ion transport, a more energetic process. Here, ions are pumped against their concentration gradient, creating a reservoir of chemical energy that powers cellular processes.
In the realm of nerve and muscle function, the ion concentration gradient is a master choreographer. It’s the force behind the electrical signals that zip through our neurons, enabling communication and control. It’s the spark that triggers muscle contractions, allowing us to move, breathe, and live.
In conclusion, the ion concentration gradient is not just a scientific concept; it’s a vital force that shapes biological processes. Understanding this gradient is key to comprehending the intricate workings of our cells and the symphony of life itself.
Permeability: The Gatekeeper of Ion Movement
Every living cell relies on the seamless movement of ions across its membranes. This intricate dance is orchestrated by an enigmatic force known as the electrochemical gradient, which drives ions from areas of high concentration to low concentration. However, the cell membrane, acting as a selective barrier, plays a crucial role in regulating this ionic flow. Its permeability—the ability to allow ions to pass through—acts as the gatekeeper, dictating the rate at which ions can traverse the membrane.
Imagine a bustling city where people are eager to move from one district to another. The membrane is like a series of gates strategically placed throughout the city, controlling the flow of people. Each gate has its own selectivity, determining which ions are allowed to pass. Some gates are wide open, allowing ions to flow freely, while others are tightly shut, restricting their passage.
The permeability of a membrane is influenced by several factors, including the size and charge of the ions, the structure of the membrane, and the presence of specific membrane proteins. These proteins, known as ion channels, act as specialized gates, selectively permitting the passage of certain ions. They can be likened to security guards, checking the credentials of each ion before granting entry.
The permeability of the membrane has a profound impact on the rate of ion movement across the membrane. A highly permeable membrane allows ions to flow rapidly, while a less permeable membrane slows their movement. This can significantly affect the rate of ion transport, which is essential for maintaining cellular homeostasis and orchestrating biological processes.
In summary, the permeability of a membrane is a crucial factor in the regulation of ion movement across cellular membranes. It acts as a gatekeeper, determining the rate at which ions can traverse the membrane. Understanding the permeability of a membrane is therefore essential for comprehending the intricate ion dynamics that underpin life itself.
Flux: Quantifying Ion Movement
In the realm of ion transport, the electrochemical gradient reigns supreme as the driving force behind ion movement across membranes. But how do we measure this net flow of ions? Enter flux, a quantitative measure that captures the essence of ion movement.
Flux Unveiled: The Essence of Ion Flow
Flux, often symbolized as J, represents the net movement of ions across a membrane. It is a vector quantity, meaning it has both magnitude and direction. The magnitude of flux indicates the rate of ion movement, while the direction specifies the net flow from a region of higher concentration to a region of lower concentration.
Unveiling the Determinants of Flux
The dance of ions across membranes is orchestrated by two key factors: the electrochemical gradient and membrane permeability. The electrochemical gradient, a combined force of ion concentration gradient and membrane potential, provides the driving force for ion movement. Membrane permeability, on the other hand, serves as the gatekeeper, determining the rate at which ions can pass through.
The Interplay of Forces: Governing Ion Flux
Flux is directly proportional to both the electrochemical gradient and permeability. The greater the electrochemical gradient, the stronger the driving force, and the faster the ion movement. Similarly, increased membrane permeability allows ions to pass more easily, resulting in higher flux.
Exploring Flux in the Context of Biological Systems
Flux plays a pivotal role in maintaining cellular homeostasis and function. In neurons, for example, the flux of ions across the membrane generates electrical signals that facilitate communication. Muscle contraction, on the other hand, relies on the flux of calcium ions into the muscle cells, triggering the release of energy and muscle movement.
Flux, a quantitative measure of ion movement, is a key indicator of the electrochemical gradient’s influence on ion transport. It not only quantifies the net flow of ions but also unveils the interplay between electrochemical forces and membrane permeability. By understanding flux, we gain insights into the fundamental processes that govern ion transport and its impact on biological systems.
Equilibrium: The Balancing Act of Ion Movement
In the dynamic world of electrochemical gradients, a delicate equilibrium exists, where the interplay of opposing forces determines the movement of ions across cell membranes. This equilibrium is a crucial balancing act between the electrochemical gradient and the membrane potential.
The electrochemical gradient represents the difference in ion concentration across a membrane and the electrical potential across that membrane. Together, these factors create a driving force that propels ions from areas of high concentration and high electrical potential to areas of low concentration and low electrical potential.
However, this movement is not unidirectional. The membrane potential, a voltage difference across the membrane, exerts an opposing force, hindering the influx of positive ions and facilitating the efflux of negative ions. This counteracting effect creates a balancing act, where the electrochemical gradient and membrane potential reach an equilibrium point.
At this equilibrium, the net movement of ions ceases, as the driving force of the electrochemical gradient is exactly counterbalanced by the opposing force of the membrane potential. This equilibrium is essential for maintaining cellular homeostasis, ensuring that ions are distributed appropriately across the membrane and that electrical signaling is properly regulated.
In summary, the equilibrium of the electrochemical gradient and membrane potential is a crucial factor in controlling ion movement across cell membranes. This delicate balance ensures that ions are transported efficiently while maintaining cellular function and electrical stability.
The Electrochemical Gradient: A Vital Force in Biological Processes
The electrochemical gradient plays a crucial role in biological systems, driving ion transport and enabling essential cellular processes. It arises from the combined effects of an ion concentration gradient and a membrane potential.
Passive Ion Transport: The Electrochemical Gradient as a Driving Force
The electrochemical gradient serves as the driving force for passive ion transport, allowing ions to move across membranes down their concentration gradient. This movement helps maintain equilibrium within cells, as ions flow from areas of high concentration to areas of low concentration.
Active Ion Transport: Overcoming Energy Barriers
In certain instances, ions need to be moved against their concentration gradient, a process known as active ion transport. This requires energy, which is derived from hydrolysis of ATP. The electrochemical gradient provides the necessary energy to drive ions uphill, facilitating critical cellular processes such as nutrient uptake and waste removal.
Nerve and Muscle Function: The Significance of Ion Movement
The electrochemical gradient plays a particularly vital role in nerve and muscle function. In nerve cells, it enables the rapid transmission of nerve impulses, as ions flow across the neuronal membrane, causing changes in membrane potential. In muscle cells, the electrochemical gradient drives the contraction and relaxation of muscle fibers, as ions move across the sarcoplasmic reticulum and plasma membrane.
The electrochemical gradient is a fundamental concept in biology, providing the driving force for ion transport across membranes. It enables passive and active ion transport, and plays a crucial role in various physiological processes, including nerve and muscle function. Understanding the electrochemical gradient is essential for comprehending the intricate workings of biological systems.