Molecular Shape Geometry: Understanding The Arrangement And Properties Of Chlorine Atoms
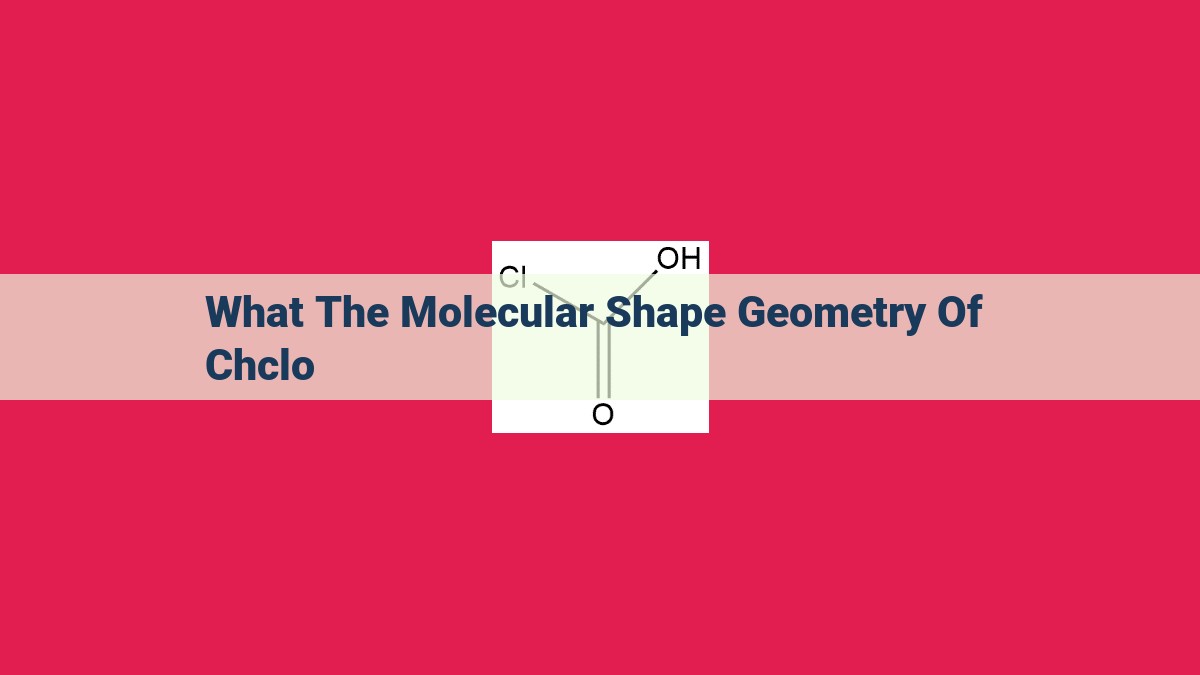
Molecular shape geometry describes the arrangement of atoms in a molecule, which determines its chemical properties. Chlorine (Cl) atoms play a crucial role in forming molecules. The tetrahedral electron domain geometry results from the arrangement of electron pairs around a central atom. Lone pair electrons, unshared by any other atom, influence molecular geometry by repelling other electron pairs. This repulsion leads to bent molecular geometry, particularly in molecules with lone pair electrons. The presence of lone pairs affects the geometry and properties of chlorine (Cl) atoms in different molecules, demonstrating the significance of molecular shape geometry in understanding molecular structure.
Molecular Shape Geometry: Delving into the World of Molecules and Chlorine Atoms
Imagine a world where atoms dance and interact, forming intricate structures that determine the properties of everything around us. One key aspect of this dance is molecular shape geometry, the arrangement of atoms in a molecule that defines its shape and behavior.
In this exploration, we will focus on chlorine (Cl) atoms and their fascinating role in shaping molecules. Chlorine, a highly reactive element, forms diverse compounds with distinct geometries, making it an ideal subject for understanding the principles of molecular shape geometry.
The Significance of Molecular Shape Geometry
Molecular shape geometry is not merely an abstract concept; it has profound implications for the properties and functions of molecules. The way atoms are arranged in a molecule influences its reactivity, polarity, solubility, and even biological activity. For instance, the shape of a drug molecule can determine its ability to interact with specific receptors in the body.
Chlorine: A Versatile Building Block
Chlorine atoms are highly versatile, participating in covalent bonds to form a wide range of molecules. These molecules exhibit various geometries, reflecting the interplay between chlorine’s valence electrons, the number of electron pairs surrounding the central atom, and the presence of lone pair electrons.
Tetrahedral Electron Domain Geometry: A Base for Understanding
To grasp the concept of molecular shape geometry, we begin with tetrahedral electron domain geometry, a fundamental arrangement where four electron pairs are distributed around a central atom. This geometry results in a tetrahedral shape, with the central atom at the center and the electron pairs forming the vertices of a tetrahedron.
Bent Molecular Geometry: The Influence of Lone Pairs
In some cases, molecules adopt a bent molecular geometry. This occurs when one or more lone pairs of electrons occupy one of the four electron domains, causing the remaining electron pairs to be pushed closer together. The resulting shape resembles a bent or V-shaped structure.
Lone Pair Electrons: Shaping the Landscape
Lone pair electrons are crucial in determining molecular shape geometry. These are pairs of electrons that do not participate in covalent bonding and occupy their own electron domains. The presence of lone pairs increases repulsion between electron domains, which in turn influences the overall shape of the molecule.
Repulsion of Lone Pair Electrons: A Balancing Act
Lone pair electrons exert a stronger repulsive force than bonding pairs of electrons. This is due to their greater concentration of electron density in a single region. The number of lone pairs and their position relative to each other determine the severity of the repulsion and the resulting molecular geometry.
Implications for Chlorine Atoms: A Case Study
Chlorine atoms exhibit various molecular shape geometries, depending on the number of electron pairs and lone pairs involved. For instance, in the molecule chlorine gas (Cl2), the two chlorine atoms form a homonuclear diatomic molecule with a linear shape. In contrast, in the molecule hypochlorous acid (HOCl), the chlorine atom adopts a bent molecular geometry due to the presence of a lone pair electron.
Tetrahedral Electron Domain Geometry
Unveiling the Three-Dimensional Architecture of Molecules
In the realm of molecular geometry, understanding the tetrahedral electron domain geometry is paramount, for it unravels the secrets of molecular shapes and their profound implications for chemical interactions.
When a central atom forms chemical bonds with four other atoms or groups of atoms, the electrons involved in these bonds and any lone pairs (unshared electron pairs) are arranged in a tetrahedral fashion. This arrangement creates a three-dimensional structure resembling a tetrahedron, a triangular pyramid with four equivalent faces.
The tetrahedral geometry arises from the repulsion between electron pairs. Electrons, being negatively charged, naturally repel each other. The tetrahedral arrangement minimizes this repulsion by maximizing the distance between electron pairs, thus achieving the most stable configuration.
Imagine a central atom surrounded by four electron pairs. Each electron pair occupies a corner of the tetrahedron, pointing away from the others. This distribution ensures that the electron pairs are as far apart as possible, resulting in the characteristic tetrahedral shape.
Bent Molecular Geometry: The Tale of Lone Pair Repulsion
Introduction
Molecular shape geometry plays a pivotal role in understanding the structure and properties of molecules. When determining molecular shape, we consider the arrangement of electron pairs around the central atom. While tetrahedral electron domain geometry is commonly observed, there are instances where molecules exhibit a bent molecular geometry.
Bent Molecular Geometry
Bent molecular geometry occurs when there are two lone pairs and two bonding pairs of electrons around the central atom. The lone pairs, which are not involved in bonding, exert a strong repulsive force on the bonding pairs. This repulsion pushes the bonding pairs closer together, resulting in a bent or V-shaped molecular geometry.
Influence of Lone Pair Electrons
The number and position of lone pairs have a profound impact on molecular geometry. The more lone pairs present, the greater the repulsion, and the more pronounced the bending of the molecule. For instance, in a molecule with two lone pairs and two bonding pairs, the lone pairs will occupy the equatorial positions, while the bonding pairs will be confined to the axial positions, resulting in a bent geometry.
Real-World Implications
Bent molecular geometry is encountered in various molecules, including water (H2O) and ammonia (NH3). In H2O, the lone pairs on the oxygen atom repel the bonding pairs, leading to a bent shape. Similarly, in NH3, the lone pair on the nitrogen atom causes a deviation from the ideal tetrahedral geometry, resulting in a trigonal pyramidal shape.
Conclusion
Bent molecular geometry is a fascinating phenomenon that arises from the interplay of electron pair repulsion and lone pair interactions. Understanding this geometry is crucial for comprehending the structure and behavior of molecules, enabling us to unravel the intricate world of chemistry.
Lone Pair Electrons: Disrupting Symmetry in Molecular Architecture
Every molecule, an intricate assembly of atoms, possesses an underlying shape determined by the arrangement of its electrons. These electrons, akin to celestial bodies, orbit the molecular nucleus, but not all are created equal. Among them reside lone pair electrons, solitary entities that exert a profound influence on molecular geometry.
Lone Pair Electrons: Their Nature and Impact
Lone pair electrons, as their name suggests, stand aloof from the bustling crowd of other electrons. They occupy molecular orbitals that are not involved in bonding with other atoms, rendering them somewhat isolated. However, their solitude does not make them passive bystanders. Lone pair electrons play an active role in shaping the geometry of molecules, wielding repulsive forces that can disrupt the otherwise harmonious symmetry.
Imagine a molecule as a miniature solar system, with the nucleus at its center. Electrons, like planets, orbit this nucleus, but some, the lone pairs, remain untethered, like celestial outcasts. Their presence creates a repulsive force field, nudging other electron pairs away from their preferred positions. This repulsion distorts the molecular geometry, leading to deviations from the ideal shapes predicted by simple models.
Implications for Molecular Shape
The presence of lone pairs can significantly alter the shape of a molecule. For instance, consider water (H2O). Without lone pairs, its molecular geometry would be linear, with the two hydrogen atoms symmetrically positioned on either side of the oxygen atom. However, the oxygen atom possesses two lone pairs, which exert a repulsive force on the hydrogen atoms. This force pushes the hydrogen atoms closer together, resulting in a bent or V-shaped molecular geometry.
The influence of lone pairs extends beyond the realm of small molecules. In complex biological molecules, such as proteins, lone pairs play a crucial role in determining the intricate folding patterns that enable these biomolecules to function. By influencing the geometry of polypeptide chains, lone pairs contribute to the formation of protein structures that are essential for life itself.
Lone pair electrons, though often overlooked, are unsung heroes in the world of molecular architecture. Their repulsive forces, like an invisible hand, shape and mold molecular geometries, giving rise to the immense diversity of chemical structures that exist in the world around us. From the simple bending of water molecules to the intricate folding of proteins, lone pairs orchestrate a dance of electrons that ultimately shapes the world we inhabit.
Repulsion of Lone Pair Electrons and Its Impact on Molecular Geometry
In the realm of molecular chemistry, the arrangement of atoms and electrons defines the structure and properties of molecules. Among the key factors that shape this molecular landscape lies the repulsion of lone pair electrons. These lone pairs, unattached electron pairs hovering around a central atom, play a crucial role in determining the geometry and behavior of the molecule.
Imagine a bustling dance floor, where electrons jostle for space around a central atom like dancers trying to find their perfect spots. Lone pair electrons, being single and unattached, are particularly prone to bumping into their neighbors. This repulsion, like a force field, pushes other electron pairs away, affecting the overall shape of the molecule.
The more lone pairs a central atom possesses, the stronger the repulsion. It’s like adding more dancers to the dance floor; the competition for space intensifies, and the jostling becomes more vigorous. This repulsion leads to a wider separation between the remaining electron pairs, influencing the molecular geometry.
For example, in a molecule like chlorine trifluoride (ClF3), the central chlorine atom has three bonding pairs and one lone pair. The repulsion from the lone pair forces the three bonding pairs to be pushed away from it, resulting in a T-shaped molecular geometry.
In contrast, a molecule like water (H2O) has two bonding pairs and two lone pairs. The stronger repulsion from the two lone pairs pushes the bonding pairs farther apart, creating a bent molecular geometry.
Understanding the repulsion of lone pair electrons is essential for predicting and explaining the diverse shapes and properties of molecules. It’s a fundamental concept that opens up a window into the intricate dance of electrons and atoms that gives rise to the chemical world around us.
Implications for Chlorine (Cl) Atoms
As we delve into the fascinating world of molecular shape geometry, chlorine (Cl) atoms emerge as key players in shaping the structure and properties of countless molecules. Their ability to form diverse bonds and accommodate lone pairs of electrons gives rise to a myriad of molecular geometries.
Tetrahedral Geometry:
In molecules like carbon tetrachloride (CCl4), chlorine atoms surround a central carbon atom in a symmetrical tetrahedral arrangement. This geometry is a consequence of the four electron pairs around the carbon atom, which repel each other to form a perfect tetrahedron.
Bent Geometry:
When chlorine atoms are bonded to a central atom with lone pairs, such as in chlorine dioxide (ClO2), a bent molecular geometry arises. The lone pairs exert repulsive forces on the bonding electron pairs, pushing them closer together and resulting in a V-shaped configuration.
VSEPR Theory:
The Valence Shell Electron Pair Repulsion (VSEPR) theory provides a framework for understanding the geometries of molecules based on the number of electron pairs around the central atom. According to VSEPR, lone pairs occupy more space than bonding pairs, leading to distortions in the molecular shape.
Impact on Molecular Properties:
The molecular shape geometry of chlorine-containing molecules has significant implications for their physical and chemical properties. For instance, the tetrahedral geometry of CCl4 contributes to its nonpolarity and high boiling point, while the bent geometry of ClO2 makes it a reactive oxidizing agent.
Chlorine atoms play a crucial role in the molecular shape geometry of countless compounds, influencing their structure, bonding, and properties. Understanding the interplay between chlorine’s electron configuration and the VSEPR theory is essential for deciphering the diverse molecular landscapes encountered in chemistry.