Understanding The Charge On Pb: Implications For Atomic Chemistry
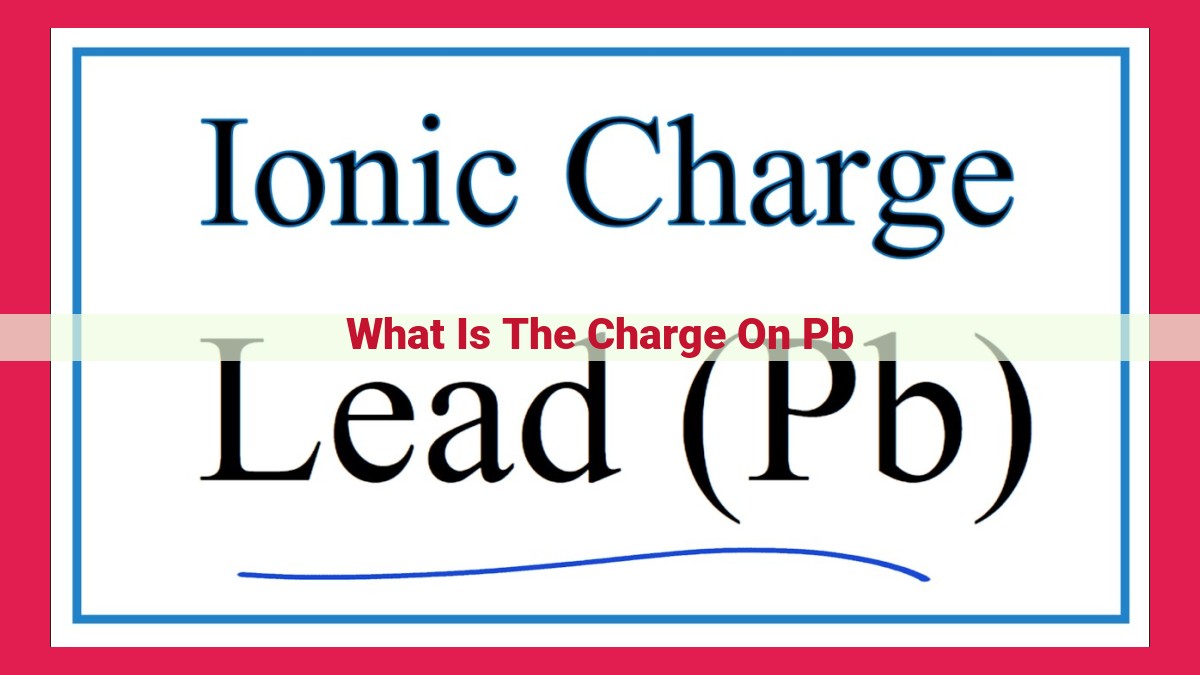
The charge on Pb is +2. Protons contribute to the positive charge of the atom’s nucleus, and the number of protons is equal to the atomic number. Since Pb has an atomic number of 82, it has 82 protons. Electrons carry negative charge, and atoms are electrically neutral when the number of electrons equals the number of protons. Pb typically loses two electrons to achieve stability, resulting in a net positive charge of +2.
Understanding Atomic Structure and Protons: The Foundation of Matter
Our world is made up of tiny building blocks called atoms, and at the heart of each atom lies its nucleus. The nucleus is where the protons reside, positively charged particles that play a crucial role in determining the identity and behavior of an element.
Protons are subatomic particles defined by their positive electrical charge and their mass. They are accompanied by neutrons, which are also found in the nucleus but carry no electrical charge. Together, the number of protons and neutrons determines the atomic number and atomic mass of an element, respectively.
The atomic number is unique to each element and represents the number of protons in its nucleus. For instance, all atoms with one proton are hydrogen, while those with six protons are carbon.
The total number of protons and neutrons in an atom determines its atomic mass. Isotopes are atoms of the same element with the same atomic number but different atomic masses. Isotopes have varying numbers of neutrons, resulting in varying atomic masses.
Electrons and Electrical Charge: Unlocking the Secrets of Atoms
In the realm of chemistry, atoms are the fundamental building blocks of matter. Within these microscopic structures lies a fascinating world of protons, neutrons, and electrons, each playing a crucial role in defining the properties of the atom. Among these, electrons hold a special significance in determining atomic neutrality and shaping the world of electricity.
Electrons, the negatively charged particles that whirl around the atomic nucleus, are the key to understanding atomic neutrality. Every atom strives to achieve a balanced electrical state, with an equal number of positively charged protons and negatively charged electrons. This equilibrium ensures that the atom remains electrically neutral, without any overall charge.
Beyond their role in maintaining neutrality, electrons also play a pivotal role in the concept of electrical charge. When atoms gain or lose electrons, they become ions, acquiring a net positive or negative charge. This phenomenon forms the basis of electrical phenomena such as conductivity.
Positive ions arise when an atom loses electrons, leaving an excess of protons. Negative ions, on the other hand, are formed when an atom gains electrons, creating an abundance of negative charges. These charged particles can freely move within conductive materials, enabling the flow of electrical current.
Understanding the nature and behavior of electrons is essential for comprehending the fundamental principles of chemistry and electricity. These tiny particles hold the key to unlocking the secrets of matter and the electrical forces that shape our world.
Polarity and Conductivity: Exploring Charge Separation and Material Behavior
In the intriguing world of atoms and molecules, where the dance of charged particles shapes their properties, we encounter the fascinating concepts of polarity and conductivity.
Polarity: A Tale of Charge Separation
Imagine a molecule as a tiny magnet, with one end positively charged and the other negatively charged. This phenomenon is known as polarity. Polarity arises when there’s an unequal distribution of electrons within a molecule, creating a separation of charge. For instance, in a water molecule, oxygen has a stronger pull on electrons than hydrogen, resulting in a slightly positive hydrogen end and a slightly negative oxygen end.
Conductivity: The Pathway for Charge Flow
Materials, like highways for charged particles, exhibit varying abilities to conduct electricity. Conductors are materials that allow electrons to flow freely through them, like a bustling city teeming with traffic. In contrast, insulators act as barriers, impeding the movement of electrons, much like a roadblock on a highway. The ability of a material to conduct electricity depends on its electronic structure and the mobility of its charge carriers (electrons or ions).
In conductors, such as metals, electrons are loosely bound to their atoms, making them free to roam the material. When a voltage is applied, these electrons can easily move in response, creating an electric current. Semiconductors, like silicon, possess an intermediate level of conductivity, while insulators, like rubber or plastic, have very limited conductivity.
The understanding of polarity and conductivity is crucial in numerous applications, from the design of electronic devices to the development of new materials. By harnessing the power of charge separation and charge flow, we unlock the potential for technological advancements that continue to shape the modern world.
Atomic Mass and Ion Formation
- Clarify atomic mass as the sum of protons and neutrons.
- Discuss how gaining or losing electrons creates ions with net charges.
Atomic Mass and Ion Formation
Understanding the fundamental building blocks of matter is crucial in chemistry. Atomic mass, a fundamental property of every element, plays a pivotal role in determining an atom’s behavior. It represents the combined weight of the protons and neutrons residing in the atom’s nucleus.
The nucleus is the dense core of an atom, housing positively charged protons and electrically neutral neutrons. The number of protons in an atom’s nucleus defines its atomic number, which uniquely identifies the element on the periodic table. Protons contribute to the atom’s overall positive charge, with their number balancing out the negative charge of electrons orbiting the nucleus.
Electrons, negatively charged particles, maintain an equal and opposite charge to the protons, resulting in an overall neutral atom. However, when atoms engage in ion formation, they can gain or lose electrons, altering their charge balance. This process results in the creation of charged particles called ions.
When an atom loses one or more electrons, it acquires a positive net charge and becomes a positively charged ion, also known as a cation. Conversely, when an atom gains one or more electrons, it accumulates a negative net charge and transforms into a negatively charged ion, known as an anion.
Ion formation is driven by the desire of atoms to achieve a stable electron configuration, typically mimicking the electronic structure of noble gases. Ions play crucial roles in countless chemical reactions, forming ionic compounds, regulating pH levels, and facilitating electrical conduction. Understanding the concept of ion formation is essential for grasping the complexities of chemical reactions and the behavior of matter.
Ions in Chemical Reactions: The Dance of Atoms
The world of chemistry is filled with fascinating transformations, and one of the most fundamental is the formation of ions. Ions are atoms that have gained or lost electrons, resulting in an imbalance between their positive and negative charges. This process plays a vital role in countless chemical reactions, shaping the molecules and materials that make up our world.
Electron Transfer: The Birth of Ions
Imagine a dance floor where atoms are the dancers. Some atoms, like sodium (Na), are eager to lose an electron, becoming positively charged cations. Others, such as chlorine (Cl), are keen on gaining an electron, transforming into negatively charged anions. This electron exchange is the foundation of ion formation.
The Role of Ions in Chemical Reactions: A Balancing Act
When ions form, they create an electrostatic attraction between themselves. This attraction drives chemical reactions between ions, leading to the formation of new compounds. For example, when sodium cations (Na+) react with chlorine anions (Cl-), they form the ionic compound sodium chloride (NaCl), commonly known as table salt.
In this reaction, sodium atoms lose an electron, becoming positively charged sodium ions (Na+), while chlorine atoms gain an electron, transforming into negatively charged chloride ions (Cl-). As these ions are drawn together by their opposite charges, they create the ionic bond that holds NaCl together.
Ions are the building blocks of countless chemical reactions, acting as the catalysts for the formation of new substances. Their unique charge properties drive their interactions with other ions, determining the structure, properties, and behavior of the molecules and materials around us. From the salt in our food to the metals in our cars, ions play an indispensable role in shaping the world we experience.
Organization of Elements in the Periodic Table: A Journey of Chemical Order
The periodic table is an ingenious arrangement of all the chemical elements known to man. It’s not just a random list but a highly structured and precise system that reveals a profound order and predictability in the world of atoms. The periodic table is the ultimate cheat sheet for chemists and scientists, and its organization based on atomic number is the key to understanding its secrets.
Atomic Number: The Identity Card of Elements
Each element in the periodic table has a unique atomic number. This number represents the number of protons (positively charged particles) in the nucleus of an atom. Protons determine an element’s position on the periodic table, as it increases from left to right across rows and from top to bottom in columns.
Groups and Periods: The Neighborhood of Elements
The periodic table is divided into vertical columns known as groups and horizontal rows called periods. Groups represent elements that share similar chemical properties because they have the same number of valence electrons (the electrons in the outermost shell). These valence electrons determine how elements react and interact with each other.
Periods, on the other hand, indicate the number of electron shells (energy levels) filled. Elements in the same period have the same number of electron shells but differ in the number of valence electrons. This affects their physical and chemical properties, revealing patterns in reactivity and other characteristics.
Groups and Valence Electrons: The Secret to Chemical Behavior
The number of valence electrons is crucial in determining an element’s chemical behavior. Elements in the same group have similar valence electrons and thus display similar chemical properties. For example, all alkali metals (Group 1) have one valence electron and are highly reactive, while all noble gases (Group 18) have eight valence electrons and are chemically inert.
Periods and Electron Shells: The Stairway to Reactivity
The periodic table also reveals patterns in reactivity based on electron shells. Elements in the same period have the same number of electron shells, but as you move from left to right across a period, the number of valence electrons increases. This affects reactivity, with elements on the left of a period generally more reactive than those on the right.
The periodic table is a testament to the intricate organization of the chemical world. Its arrangement based on atomic number, groups, and periods provides a roadmap for understanding the properties and behaviors of elements. By delving into the periodic table’s structure, chemists and scientists can unlock the secrets of the material world and harness the power of elements to create new materials, advance technologies, and improve our lives.
Group Properties: Valence Electrons
In the captivating tale of the periodic table, groups, like chapters in a grand novel, gather elements with similar chemical quirks. This kinship stems from a secret shared among these elemental neighbors: valence electrons. These outermost electrons, like the lively characters in a play, determine an element’s penchant for chemical interactions.
Valence electrons are the key players in the dance of reactivity. They are the electrons that dance freely around the atomic nucleus, eager to mingle with others. The number of valence electrons is akin to the social circle an element keeps. Elements with more valence electrons are more outgoing and interactive, while those with fewer are more reserved.
Consider the alkali metals, a gregarious group that inhabits the far left column of the periodic table. Each alkali metal atom has a single valence electron, like a solitary extrovert itching to connect. This lone electron makes them highly reactive, as they eagerly donate it to form chemical bonds.
In contrast, the noble gases reside on the opposite end of the spectrum, their valence shells filled to the brim. Like solitary introverts, they prefer their own company and rarely participate in chemical reactions. Their lack of valence electrons makes them chemically inert, hence their nickname “inert gases.”
The halogens, on the other hand, are a cunning bunch. They have seven valence electrons, just one short of a full shell. This makes them eager to acquire one more electron to complete their set. As a result, halogens are highly reactive, forming compounds with almost every other element.
Understanding the concept of valence electrons is crucial for comprehending the diverse chemical behaviors of elements. It’s like having a secret code that unravels the mysteries of chemical reactions, allowing us to predict how elements will interact and form the building blocks of our world.
Period Properties: Electron Shells
As we journey through the periodic table, the arrangement of elements isn’t merely a coincidence. The horizontal rows, known as periods, represent a profound pattern in the atomic structure of elements. Each period unveils the number of complete electron shells an element possesses.
Elements within the same period share a unique trait: they all have an equal maximum number of electrons that can occupy their outermost shell. This outermost shell, known as the valence shell, plays a crucial role in determining an element’s chemical behavior.
Consider the first period of the periodic table, consisting of hydrogen (H) and helium (He). Both H and He share a common feature: they have only one electron shell. H’s valence shell is not complete, with only one electron, while He has a complete valence shell with two electrons. This difference in valence electrons accounts for their distinct chemical properties.
As we move across the periodic table, the number of electron shells increases. Elements in the second period possess two electron shells. This includes elements like lithium (Li), beryllium (Be), and boron (B). Again, while Li has a single valence electron, Be and B have two and three valence electrons, respectively.
The trend continues as we delve deeper into the periodic table. Period 3 elements, such as sodium (Na), magnesium (Mg), and aluminum (Al), boast three electron shells and exhibit a range of valence electrons.
This pattern underscores the significance of electron shells in understanding the chemical properties of elements. Elements within the same period share similar outermost electron configurations, leading to predictable trends in their reactivity and bonding behaviors. By comprehending the concept of electron shells, we unlock a deeper appreciation for the intricate organization within the periodic table.