Unveiling Carbon’s Versatility: Understanding Tetravalence And Covalent Bonding
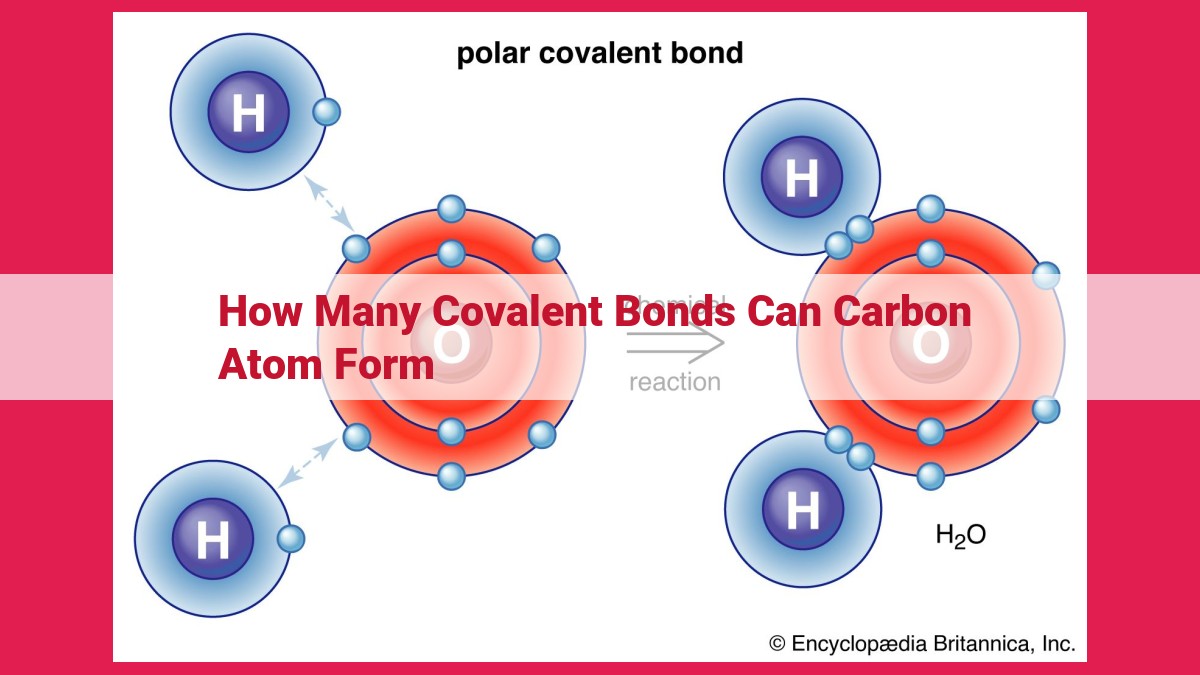
Carbon, with its four valence electrons, has the ability to form four covalent bonds. This tetravalence results from sp3 hybridization, which creates four equivalent hybrid orbitals with tetrahedral geometry. These orbitals overlap with orbitals from other atoms, forming covalent bonds. Carbon can also form double and triple bonds by sharing multiple pairs of bonding electrons. This versatility in bonding enables carbon to form a wide range of compounds, including the various allotropes of diamond, graphite, and fullerenes.
Tetravalence: The Foundation of Carbon’s Bonding
Carbon, the Versatile Building Block of Life
In the realm of chemistry, carbon stands as a remarkable element, renowned for its versatility and ability to form a vast array of molecules. At the heart of carbon’s bonding prowess lies its tetravalence, a fundamental property that enables it to form up to four covalent bonds with other atoms.
Four Valence Electrons: A Recipe for Bonding
Each carbon atom possesses four valence electrons, nestled in its outermost energy level. These electrons, eager to bond with others, are the driving force behind carbon’s remarkable bonding capacity. By sharing these electrons with other atoms, carbon can create stable and robust covalent bonds.
Hybridization: Creating the Perfect Bonding Geometries
To form these covalent bonds, carbon employs a clever strategy known as hybridization. It merges its atomic orbitals, creating new hybrid orbitals with specific shapes and orientations. The most common hybridization in carbon is sp3 hybridization, which gives rise to four equivalent hybrid orbitals arranged in a tetrahedral geometry. This arrangement provides the ideal platform for carbon to bond with four other atoms.
Orbital Overlap: The Key to Bonding
The formation of a covalent bond between carbon and another atom occurs through a process known as orbital overlap. The hybrid orbitals of carbon overlap with the orbitals of the other atom, creating a region of electron density shared between the two atoms. This overlap ensures that the electrons are effectively shared, forming a strong and stable bond.
Tetravalence, hybridization, and orbital overlap are the key concepts that underpin carbon’s bonding prowess. By understanding these principles, we gain a deeper appreciation for the intricate dance of electrons that creates the molecular building blocks of our world. From the countless organic compounds that form the basis of life to the versatile materials that shape our technologies, carbon’s tetravalence is a fundamental driver of chemical diversity and innovation.
Hybridization: Creating Hybrid Orbitals for Bonding
In the intriguing world of chemistry, carbon reigns supreme as the “King of Elements” due to its unparalleled ability to form diverse and complex molecules. At the heart of this extraordinary capability lies a fundamental concept known as hybridization.
Hybridization: A Tale of Atomic Transformation
Imagine a carbon atom, a tiny sphere brimming with four valence electrons eager to interact with the world around them. These electrons reside in different orbitals, each resembling a hazy cloud surrounding the atomic nucleus.
When carbon seeks to form bonds, these individual orbitals undergo a remarkable transformation called hybridization. During this process, they merge their identities, blending their shapes and energies to create new hybrid orbitals.
Sp3 Hybridization: The Tetrahedral Dance
The most prevalent type of hybridization experienced by carbon is known as sp3 hybridization. Picture four hybrid orbitals emerging from the carbon atom like the arms of a tetrahedron, a geometric shape with four triangular faces. These hybrid orbitals are identical in shape, energy, and orientation, forming a symmetrical tetrahedral configuration.
The magic of sp3 hybridization lies in its ability to accommodate four electron pairs, each of which can form a covalent bond with another atom. This tetrahedral arrangement gives rise to the characteristic three-dimensional structure of many organic molecules, the building blocks of life itself.
The Importance of Hybridization
Hybridization is the cornerstone of carbon’s versatility. It enables the formation of stable, covalent bonds in various orientations, leading to the creation of a vast array of molecules. This phenomenon underpins the structure and properties of countless substances, from the fuel that powers our vehicles to the DNA that carries our genetic heritage.
Delving into the World of Carbon Chemistry
To fully appreciate the significance of hybridization, it is essential to dive into the wider realm of carbon chemistry. Explore the intriguing world of allotropes, where carbon atoms arrange themselves in distinct patterns to form substances as diverse as diamond, graphite, and fullerenes. Each allotrope possesses unique properties due to the subtle variations in their covalent bonding arrangements.
By unraveling the mysteries of hybridization, we gain a profound understanding of carbon’s chemistry and its pivotal role in the intricate tapestry of life.
Orbital Overlap: The Covalent Bond’s Secret
Picture two lonely atoms floating in the vastness of space. Each atom has its own atomic orbitals, swirling clouds of electrons. But these atoms have an irresistible attraction to each other, a magnetic pull that draws them together.
As they approach, their atomic orbitals begin to overlap, intertwining like fingers. This overlap creates a new space where the electrons from both atoms can coexist. It’s a shared space, a common playground where electrons can frolic and dance.
Within this shared space, the electrons form covalent bonds. These bonds are the glue that holds atoms together, creating the building blocks of matter. It’s like a molecular handshake, where each atom’s electrons hold on tightly to the other atom’s electrons, forging an unbreakable bond.
The strength of the covalent bond depends on how much the atomic orbitals overlap. The greater the overlap, the stronger the bond. It’s a testament to the power of sharing, as the electrons in the overlapping orbitals find stability and belonging in their new, united space.
This orbital overlap is the secret ingredient in the world of chemistry. It’s the key that unlocks the door to understanding the countless molecules that make up our world. Carbon, with its tetravalence and sp3 hybridization, shines as a prime example, forming a myriad of compounds with its ability to overlap its orbitals and share electrons.
Lone Pairs: A Rare Phenomena in Carbon’s Covalent Dance
In the world of covalent bonding, carbon stands out as a maestro, orchestrating a symphony of shared electrons with its four valence electrons. However, amidst this harmonious melody, there’s a notable silence: lone pairs, those unpaired electrons seeking solitude.
In carbon’s stable covalent compounds, lone pairs are like shy dancers that prefer to stay on the sidelines. Unlike other elements that flaunt lone pairs, carbon keeps its electrons paired up, forming strong and stable bonds. This unique characteristic stems from carbon’s tetravalence, its ability to form four covalent bonds.
When carbon atoms bond with other atoms, they undergo a magical transformation, a process called hybridization. This alchemy creates hybrid orbitals, new and improved versions of atomic orbitals that blend together to form electron clouds with specific shapes.
In the case of carbon, sp3 hybridization reigns supreme, resulting in four tetrahedral hybrid orbitals that point towards the corners of a tetrahedron. These hybrid orbitals eagerly seek partners, overlapping with orbitals from other atoms to forge covalent bonds.
And as if guided by an invisible hand, the electrons pair up, cohabitating in molecular orbitals that embrace both atoms. This covalent bonding dance fills every orbital, leaving no lone pairs in sight.
Carbon’s aversion to lone pairs ensures that its covalent bonds are strong and stable. These bonds form the backbone of countless organic molecules, the building blocks of life and countless materials that shape our world.
So, while other elements may indulge in the allure of lone pairs, carbon remains steadfast in its pursuit of covalent harmony, a testament to its exceptional bonding prowess.
Double and Triple Bonds: Enhanced Covalent Interactions
Carbon’s versatility extends beyond single covalent bonds. It can also form double bonds and triple bonds through the sharing of multiple pairs of bonding electrons. This enhanced bonding capacity allows carbon to stabilize various molecular structures and expand its chemical repertoire.
In a double bond, two pairs of bonding electrons are shared between two carbon atoms. This creates a stronger bond than a single bond and results in sp2 hybridization. This hybridization involves the mixing of one 2s orbital with two 2p orbitals, resulting in three equivalent hybrid orbitals that form a trigonal planar arrangement. The remaining 2p orbital remains unhybridized and participates in the formation of the double bond.
Triple bonds are even more robust than double bonds. They involve the sharing of three pairs of bonding electrons between two carbon atoms. This necessitates sp hybridization, which combines one 2s orbital with one 2p orbital. The resulting two equivalent hybrid orbitals form a linear arrangement, while the remaining two 2p orbitals are unhybridized and involved in the formation of the triple bond.
Double and triple bonds are crucial in the formation of various carbon-based molecules, including organic compounds. They enhance the stability and reactivity of these molecules and contribute to the diverse properties of carbon. From the rigid structure of diamond to the flexible sheets of graphite, these enhanced covalent interactions play a pivotal role in shaping the molecular world of carbon.
Allotropes: Carbon’s Versatile Bonding Manifestations
Carbon, an element with a mere six protons, is renowned for its exceptional versatility, manifesting in a kaleidoscope of allotropes—distinct forms that showcase the element’s diverse bonding capabilities.
Diamond: The Hardest Natural Substance
Diamond, a symbol of enduring love, owes its unparalleled hardness to its tightly packed crystalline structure. Each carbon atom in a diamond forms four covalent bonds with its neighbors, creating a rigid three-dimensional network that resists deformation. This exceptional bonding arrangement renders diamond the hardest naturally occurring substance known to humankind.
Graphite: The Conductive Black
Graphite is the polar opposite of diamond in terms of physical properties. Its layered structure, composed of sheets of carbon atoms arranged in hexagonal rings, results in weak inter-layer bonding. This unique bonding arrangement grants graphite its characteristic softness and electrical conductivity, making it the material of choice for pencil electrodes and electrical components.
Fullerenes: Hollow Carbon Spheres
Fullerenes are carbon molecules shaped like hollow spheres, ellipsoids, or tubes. These unique structures arise from the curvature of carbon-carbon bonds, which creates a highly strained yet stable framework. Fullerenes have garnered interest for their potential applications in nanotechnology and medicine.
The array of carbon allotropes underscores the element’s remarkable bonding versatility. From diamond’s impenetrable hardness to graphite’s electrical conductivity and fullerenes’ novel shapes, the bonding of carbon atoms gives rise to a spectrum of materials with astonishing properties. Understanding the bonding of carbon is fundamental to unraveling the mysteries of life and harnessing the power of this versatile element for technological advancements.