Understanding Active Vs. Passive Transport: Atp Vs. Concentration Gradient
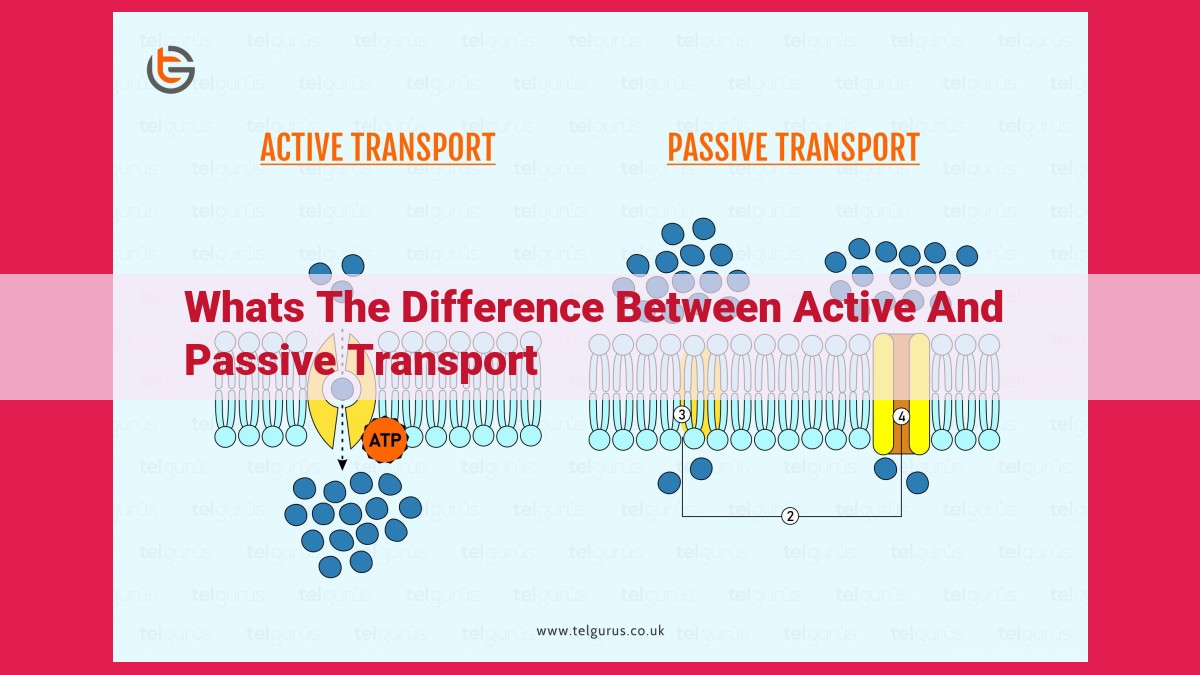
Active vs. Passive Transport: Active transport defies concentration gradients, requiring ATP to move substances against their gradient. Passive transport, driven by concentration gradients, moves substances down their gradient, requiring no energy input.
Understanding Active vs. Passive Transport: The Cellular Balancing Act
In the world of microscopic wonders, where cells constantly buzz with activity, the movement of molecules plays a vital role. Understanding how this molecular traffic operates is essential for comprehending the inner workings of life.
Enter active and passive transport—two fundamental modes of molecular movement across cell membranes. These processes are as different as day and night. Active transport fights against the odds, valiantly pumping molecules uphill against a concentration gradient. It’s like a miniature tugboat, defying the natural flow of molecules to create and maintain essential cellular gradients. The secret weapon of active transport? ATP, the cell’s energy currency, which fuels this uphill struggle.
In contrast, passive transport rides the wave of nature’s gradients, allowing molecules to glide effortlessly downhill from high to low concentrations. It’s like a lazy river ride, where molecules float along the current without any energy expenditure. Diffusion and osmosis—two forms of passive transport—ensure that life’s building blocks distribute evenly throughout the cell.
The driving force behind molecular movement is the concentration gradient, a measure of the difference in concentration of a substance across a membrane. These gradients act like invisible magnets, pulling molecules from areas of high concentration to low concentration. As gradients dissipate, molecules reach equilibrium, a state of balance where their distribution throughout the cell is uniform.
Facilitated diffusion, a special case of passive transport, enlists the help of membrane proteins to ferry molecules across the membrane. These proteins act like molecular gatekeepers, allowing only specific molecules to pass, ensuring the selective entry and exit of critical substances.
Understanding these transport mechanisms is not merely an academic exercise. It holds profound implications for understanding how cells maintain homeostasis, the delicate balance of internal conditions essential for life. The intricate dance of active and passive transport, along with the concept of concentration gradients and equilibrium, orchestrates the cellular symphony, keeping life’s microscopic engines humming along.
Active Transport: Defying the Gradient
In the realm of cellular transport, active transport stands as a remarkable feat of biological defiance. Unlike passive transport, which relies on the natural flow of molecules down concentration gradients, active transport propels molecules against this gradient, creating and maintaining crucial differences in concentration across cell membranes.
ATP: The Fuel of Active Transport
The energy required for this uphill battle is supplied by a cellular powerhouse: adenosine triphosphate (ATP). This high-energy molecule serves as the primary currency of energy within cells. When ATP is hydrolyzed, releasing energy, it fuels the membrane proteins responsible for pumping molecules across the gradient.
Maintaining Concentration Gradients
By actively transporting molecules against the natural flow, cells create and maintain concentration gradients. These gradients are essential for various cellular processes, including nutrient uptake, waste removal, and the establishment of electrical signals.
Examples of Active Transport
A myriad of substances are transported against their gradients via active mechanisms, including:
- Sodium ions (Na+) and potassium ions (K+): These ions are crucial for maintaining the electrical potential across cell membranes and facilitating nerve impulses.
- Calcium ions (Ca2+): Calcium ions play a vital role in muscle contraction, cellular signaling, and bone formation.
- Glucose: This sugar molecule is transported into cells against a concentration gradient to provide energy.
Active transport is a remarkable feat of cellular biology. By defying concentration gradients with the help of ATP, cells can create and maintain essential differences in molecular distribution. This process plays a pivotal role in countless cellular processes, showcasing the incredible power and adaptability of life’s molecular machinery.
Passive Transport: Nature’s Balancing Act
Our cells are constantly interacting with their surroundings, exchanging various substances to maintain their internal balance. Passive transport is a fundamental process that enables this vital exchange without requiring any energy input from the cell.
Diffusion: Molecules on the Move
Diffusion is the random movement of molecules from areas of high concentration to areas of low concentration. It occurs when there’s an uneven distribution of particles. Imagine a crowded room where everyone is trying to get to the door at the same time. Molecules behave similarly, spreading out from areas where they’re densely packed to areas where they’re less concentrated.
Osmosis: Water’s Selective Dance
Osmosis is a specialized form of diffusion where water molecules move across a semipermeable membrane. This membrane acts like a filter, allowing water molecules to pass through but blocking larger particles. When water concentration is higher on one side of the membrane, it flows to the side with a lower concentration, balancing out the water levels.
Importance of Passive Transport
Passive transport plays a crucial role in various cellular functions:
- Nutrient uptake: Cells absorb essential nutrients, such as glucose and amino acids, through passive transport.
- Waste removal: Cells eliminate waste products, such as carbon dioxide and urea, by diffusion and osmosis.
- Maintaining cell volume: Osmosis helps regulate cell volume by preventing water from entering or leaving too quickly.
Passive transport is a fundamental mechanism that allows our cells to function efficiently and maintain homeostasis, the delicate balance of their internal environment. It’s a testament to nature’s ingenuity, using simple yet effective processes to support life’s intricate machinery.
Concentration Gradient: The Driving Force
- Explain the concept of a concentration gradient and its role in both active and passive transport.
- Discuss how equilibrium is established when concentration gradients disappear.
- Provide examples of concentration gradients in biological systems.
Concentration Gradient: The Driving Force of Molecular Transport
Imagine a bustling city where molecules are like tiny citizens, constantly in motion. There’s a constant flow of molecules in and out of cells, organs, and tissues, and the direction of this flow is determined by something called a concentration gradient.
A concentration gradient is the difference in the amount of a substance between two areas. It’s like a magnet, drawing molecules from areas of high concentration to areas of low concentration, like water flowing downhill. This gradient is the driving force behind both active and passive transport, the two types of molecular transport that keep our bodies functioning.
In active transport, molecules are moved against the concentration gradient, from areas of low concentration to areas of high concentration. This requires energy, which is typically provided by ATP, the energy currency of cells. Examples of active transport include the sodium-potassium pump and the glucose transporter in our cell membranes.
In passive transport, molecules move with the concentration gradient, from areas of high concentration to areas of low concentration. This doesn’t require energy input, which is why it’s called passive. Diffusion and osmosis are two common types of passive transport.
Diffusion is the movement of molecules from areas of high concentration to areas of low concentration, like a drop of food coloring spreading in a glass of water. Osmosis is a specific type of diffusion that involves the movement of water across a selectively permeable membrane, from areas of high water concentration to areas of low water concentration.
Concentration gradients play a crucial role in maintaining equilibrium, a state of balance where the concentration of molecules is the same in all areas. When concentration gradients disappear, equilibrium is established and the flow of molecules stops.
In biological systems, concentration gradients are found throughout the body. For example, the concentration of oxygen is higher in the lungs than in the blood, creating a gradient that drives oxygen into the bloodstream. The concentration of glucose is higher in the blood than in cells, creating a gradient that drives glucose into cells for energy production.
Understanding concentration gradients is essential for understanding how molecules move in and out of cells and how our bodies function. It’s a fundamental concept in biology that helps us appreciate the intricate dance of molecules that keeps us alive.
Equilibrium: A State of Balance in Molecular Transport
In the realm of molecular transport, equilibrium is a state of harmony, where the continuous waltz between active and passive transport reaches a standstill. It occurs when the forces driving molecular movement, such as concentration gradients, are perfectly balanced, resulting in an absence of net movement.
Imagine a crowded dance floor, where individuals are constantly shuffling around, bumping into each other. As the night progresses, the crowd gradually thins out until eventually, a state of equilibrium is reached. At this point, the number of individuals entering and leaving the dance floor is equal, creating a perfect balance.
Similarly, in the cellular world, equilibrium is attained when the concentration gradient that drives molecular movement disappears. This occurs when the concentration of a substance is the same on both sides of a semi-permeable membrane, such as the cell membrane. When this balance is achieved, the relentless flow of molecules from areas of high concentration to low concentration ceases.
Equilibrium plays a crucial role in maintaining cellular homeostasis, the delicate balance that keeps cells functioning optimally. Without equilibrium, essential substances would not be able to enter or leave cells, disrupting cellular processes and potentially leading to cell death. For example, equilibrium ensures that cells maintain a constant intracellular concentration of sodium and potassium ions, which is critical for nerve impulse transmission and muscle contraction.
In summary, equilibrium is a state of balance in molecular transport, where the forces driving movement are perfectly counteracted. It is essential for maintaining cellular homeostasis and ensuring the proper functioning of cells and organisms as a whole.
Facilitated Diffusion: Passive Transport with a Helper
When we think of passive transport, we often picture molecules effortlessly moving down their concentration gradients, from high to low, without any energy input. However, there are certain situations where these molecules need a helping hand to cross a biological membrane. Enter facilitated diffusion, a subtype of passive transport that provides molecular movement assistance without compromising the principle of down-the-gradient movement.
Facilitated diffusion relies on the cooperation of membrane proteins that act as gatekeepers, allowing specific molecules to pass through the membrane without disrupting its integrity. These proteins have special channels or pores that accommodate certain molecules, ensuring their efficient passage. Think of them as molecular concierges, guiding guests (molecules) through a crowded lobby (membrane).
The key distinction of facilitated diffusion from other passive transport mechanisms is the carrier protein. Unlike diffusion and osmosis, which allow molecules to move freely through the membrane, facilitated diffusion requires the assistance of these protein helpers. This controlled movement ensures that only specific molecules, those that fit the concierge’s criteria, are allowed to cross.
Although facilitated diffusion still operates down a concentration gradient, it skips the energy expense of active transport. Molecules move smoothly from an area of higher concentration to lower concentration without any ATP investment. This process is particularly crucial for transporting larger molecules or ions that cannot navigate the membrane on their own.
Facilitated diffusion has its advantages, but it also faces limitations. It is a highly specific process, meaning each carrier protein can assist only with certain molecules. This specificity can be both an advantage, ensuring selective permeability, and a disadvantage, limiting the transport options for other molecules.
Furthermore, facilitated diffusion can become saturated when the concentration of molecules exceeds the capacity of the available carrier proteins. Ima