Understand Cellular Respiration: Converting Glucose Into Cellular Energy
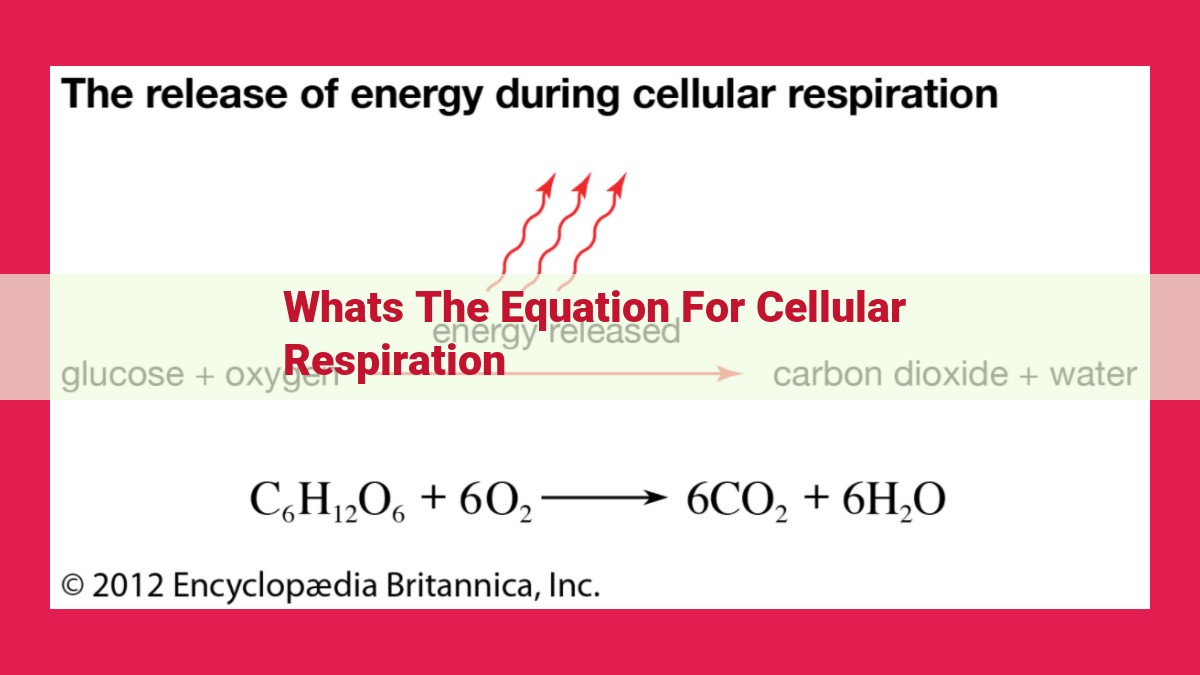
Cellular respiration is a complex process that converts glucose into energy-rich ATP molecules. The equation for this process is:
C6H12O6 + 6O2 → 6CO2 + 6H2O + 38ATP
This equation represents the breakdown of glucose (C6H12O6) in the presence of oxygen (6O2) into carbon dioxide (6CO2), water (6H2O), and a net yield of 38 ATP molecules. The ATP molecules serve as the primary energy currency for cellular activities, powering various physiological processes.
Decoding Cellular Respiration: The Fuel that Powers Life
In the intricate machinery of life, cellular respiration stands as the unsung hero, the energy-generating powerhouse that sustains every living organism. This intricate dance of biochemical reactions transforms glucose into usable energy, fueling our every move, thought, and breath.
At the heart of cellular respiration lies a chemical equation that embodies its profound significance:
C6H12O6 (Glucose) + 6O2 (Oxygen) → 6CO2 (Carbon Dioxide) + 6H2O (Water) + Energy (as ATP)
This seemingly simple equation conceals a complex symphony of interconnected reactions. It represents the journey of glucose, a sugar molecule, as it undergoes a meticulously orchestrated breakdown to release energy. The energy, harnessed in the form of ATP (adenosine triphosphate), serves as the cellular currency that powers our biological processes.
Cellular respiration is a symphony of three distinct stages: glycolysis, the Krebs cycle (also known as the citric acid cycle), and the electron transport chain. Each stage plays a vital role in extracting the maximum energy from glucose. Let’s delve into each stage, unraveling its intricacies.
Glycolysis: The Initial Breakdown in Cellular Respiration
In the fascinating realm of cellular respiration, the process of breaking down glucose for energy kicks off with a crucial stage known as glycolysis. This intricate pathway, also known as the Embden-Meyerhof-Parnas pathway, marks the initial breakdown of glucose, the body’s primary fuel source.
During glycolysis, a single molecule of glucose embarks on a mesmerizing journey, undergoing a series of 10 enzymatic reactions. Like a carefully orchestrated dance, these reactions gradually split the glucose molecule into two molecules of pyruvate.
As glucose embarks on its metabolic adventure, it encounters a crucial intersection where two molecules of ATP are invested into the process. This seemingly counterintuitive step is like a smart investment, as it sets the stage for an energy payoff later on. With these two ATP molecules invested upfront, glycolysis can proceed with its transformative work.
The end result of glycolysis is the creation of two pyruvate molecules, each carrying three carbon atoms. Additionally, the pathway generates a net gain of two ATP molecules and two molecules of NADH, a high-energy electron carrier. These energy carriers will play a pivotal role in the subsequent stages of cellular respiration, where the real energy payoff awaits.
Glycolysis, therefore, serves as the foundational stage of cellular respiration, preparing the glucose molecule for further breakdown and energy extraction. It is the initial breakdown, laying the groundwork for the subsequent steps that will ultimately generate the ATP molecules that power our cells.
The Krebs Cycle: Unleashing Energy from Pyruvate
In the heart of cellular respiration, where energy is forged, lies the intricate Krebs cycle, also known as the citric acid pathway. This metabolic dance orchestrates the breakdown of pyruvate, a product of glycolysis, to yield precious energy carriers that fuel the cell.
The Krebs cycle, an eight-step biochemical ballet, resides within the cellular powerhouse, the mitochondria. Here’s how this dance of energy unfolds:
- Acetyl-CoA Enters the Cycle: The journey begins with acetyl-CoA, the product of pyruvate breakdown, joining the cycle as acetyl-CoA combines with oxaloacetate to form citrate.
- Oxidation and Energy Extraction: Citrate undergoes a series of chemical transformations, releasing carbon dioxide (CO2) and generating NADH and FADH2, high-energy electron carriers that store the liberated energy.
- Regeneration of Oxaloacetate: The cycle completes when oxaloacetate is regenerated from malate, providing a foundation for the next round of acetyl-CoA entry and energy extraction.
Through the Krebs cycle’s rhythmic movements, pyruvate is stripped of its remaining energy, yielding a bountiful harvest of NADH and FADH2. These electron carriers, brimming with captured energy, embark on their next mission, fueling the electron transport chain and ultimately generating the cellular energy currency, ATP.
The Electron Transport Chain: The Powerhouse of ATP
In the realm of cellular respiration, the electron transport chain (ETC) stands as the mighty powerhouse, churning out the fuel that powers the body’s energy demands. This intricate network of proteins and electron carriers plays a pivotal role in the final stage of respiration, orchestrating the generation of the cellular energy currency: ATP.
The ETC is a veritable dance floor for electrons, where they skip merrily from one carrier protein to the next, like excited partygoers passing a spark around. As each electron makes its way down this electron slide, it releases a tiny burst of energy, akin to a spark igniting a flame. This energy is captured and used to pump protons from the mitochondrial matrix to the intermembrane space, creating a proton gradient like a miniature dam.
Oxidative phosphorylation, the biological name for this proton-pumping party, is a vital process that cranks out ATP. As protons flow back down their gradient through a protein called ATP synthase, they collide with spinning rotors, causing them to twirl and release precious ATP molecules. This molecular ballet produces a whopping 32 ATP molecules per glucose molecule, providing a potent energy source that fuels all aspects of cellular activity.
ATP: The Cellular Energy Currency
The Energy Powerhouse of Every Cell
Cellular respiration, the process by which cells generate energy, is like a complex symphony of biochemical reactions. And at the heart of this symphony lies a molecule called ATP (adenosine triphosphate). It’s the cellular energy currency, the vital fuel that powers every aspect of our cells’ activities.
ATP’s Role: The Catalyst of Energy Exchange
Think of ATP as the spark that ignites cellular processes. It’s a molecule that stores energy in its chemical bonds. When these bonds are broken, energy is released, powering the various functions of cells, such as muscle contraction, nerve impulses, and nutrient transport. Cells constantly break down and regenerate ATP to meet their energy demands.
The Chemiosmotic Hypothesis: Harvesting Energy from Gradients
The process of ATP generation is known as oxidative phosphorylation. It’s a complex mechanism that involves the chemiosmotic hypothesis, a theory that explains how cells harness energy by creating a gradient of ions across their membranes. This gradient is then utilized by an enzyme called ATP synthase to generate ATP.
ATP Synthase: The Molecular Machine
ATP synthase is a remarkable protein complex embedded in mitochondrial membranes. It acts like a tiny turbine, driven by the flow of ions across the membrane. This flow spins the rotor of ATP synthase, which then drives the synthesis of ATP from ADP (adenosine diphosphate) and inorganic phosphate.
The Importance of ATP
ATP is the universal energy carrier in all living organisms. It’s essential for virtually every cellular process. Without it, cells would be unable to perform their functions, and life as we know it would cease to exist. Understanding the role of ATP in cellular respiration helps us appreciate the intricate and highly efficient system that sustains all biological activity.
Key Molecules in Cellular Respiration: The Players in the Energy Equation
In the intricate symphony of life, cellular respiration stands as the maestro, orchestrating the conversion of food into the energy that fuels our cells. Among the many molecules that play vital roles in this process, a few stand out as essential players.
Glucose: The fuel that kicks off the process, glucose is the primary energy source for cellular respiration. Through a series of intricate steps, glucose is broken down to release its stored energy.
Oxygen: Acting as the final electron acceptor, oxygen plays a critical role in the last stage of cellular respiration, the electron transport chain. Without oxygen, this vital process would stall, leaving cells starved of energy.
Carbon Dioxide: A byproduct of cellular respiration, carbon dioxide represents the remnants of glucose after its energy has been extracted. This waste product is released into the bloodstream to be exhaled.
Water: A humble yet indispensable molecule, water participates throughout cellular respiration, from the breakdown of glucose to the synthesis of ATP. Its versatility makes it a crucial ingredient in the energy-producing machinery of cells.
NADH and FADH2: These electron carriers, NADH and FADH2, act as energy shuttles, capturing electrons released during the breakdown of glucose and passing them on to the electron transport chain, driving the production of ATP.
ATP: The end product of cellular respiration, ATP, is the universal energy currency of cells. Its high-energy bonds store the energy harnessed from glucose, allowing cells to perform a myriad of essential functions, from contracting muscles to synthesizing proteins.
Importance of Understanding the Cellular Respiration Equation
Comprehending the cellular respiration equation is akin to unlocking a treasure trove of knowledge about how life harnesses energy. It provides a comprehensive roadmap of the intricate biochemical processes that sustain every living organism.
By delving into the equation, we gain insights into the remarkable complexity of energy metabolism. It reveals the intricate interplay of molecules and pathways that culminate in the production of ATP, the universal energy currency of cells. Each step, from glycolysis to the Krebs cycle and electron transport chain, demonstrates the meticulous choreography of life’s molecular machinery.
Moreover, understanding the equation fosters an appreciation for the remarkable efficiency of energy metabolism. The equation deftly quantifies the precise amount of energy released from glucose, the fundamental molecule that fuels life. It highlights how cells have evolved to maximize energy extraction while maintaining a delicate balance with their environment.
In essence, the cellular respiration equation is not merely a collection of symbols, but a profound testament to the intricate and efficient mechanisms that sustain life. It provides a fundamental framework for understanding how organisms harness energy to power their biological processes.