The Ultimate Guide To Water Electrification: Electrolysis, Triboelectric, Piezoelectric, And Photoelectric Effects
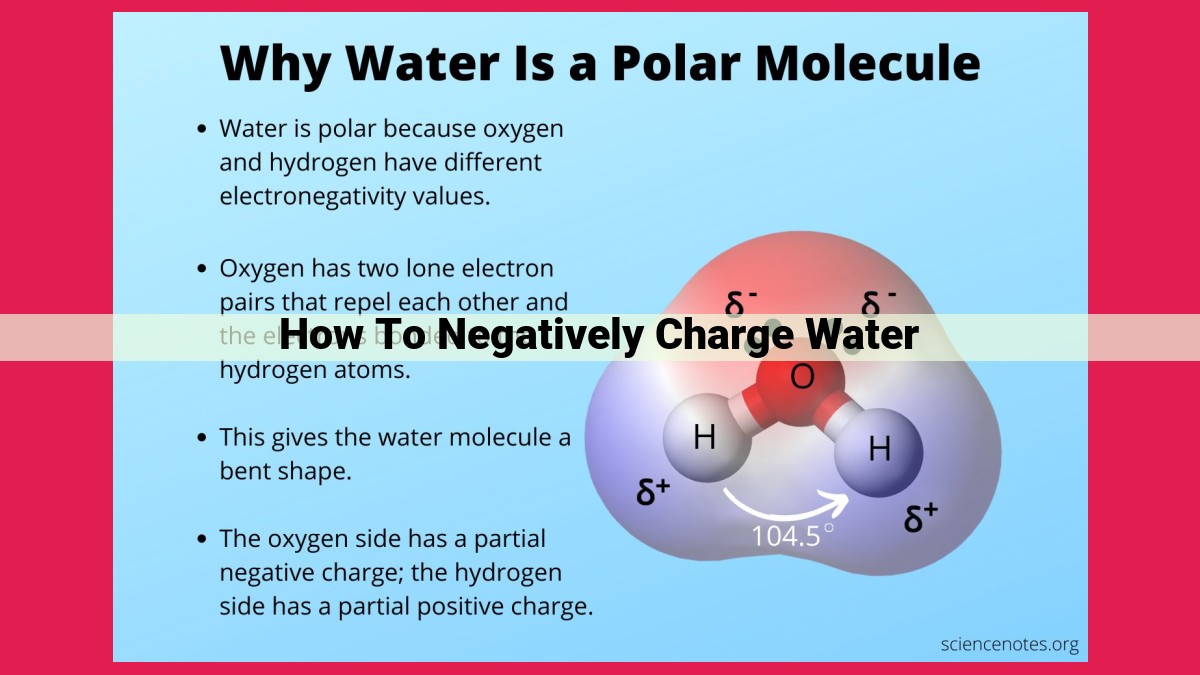
To negatively charge water, electrolysis breaks water down into hydrogen and oxygen, creating a charge imbalance. Alternatively, the triboelectric effect transfers electrons from a material to water droplets when they’re brought into contact. Piezoelectricity generates charges in water under mechanical stress, while the photoelectric effect does so using light.
The Electric Nature of Water: Unraveling the Secrets of Charging H2O
Water, the lifeblood of our planet, is often perceived as a neutral entity lacking any electrical properties. However, beneath this seemingly unassuming facade lies a hidden realm of electrochemistry, where water’s true nature as a versatile electrical conductor is revealed.
Understanding Water’s Neutrality
In its purest form, water exists as a neutral molecule, with an equal number of positively charged protons and negatively charged electrons. This balance results in a net zero charge, making water electrically neutral. However, this neutrality is not absolute and can be manipulated through various processes, revealing the diverse electrical capabilities of water.
Electrolysis: Breaking Down Water with Electricity
Electrolysis, a fascinating process that harnesses the power of electricity, decomposes water molecules into their constituent elements, hydrogen, and oxygen. When an electric current is passed through water, it splits the water molecules apart, allowing the hydrogen ions to migrate towards the negative electrode (cathode) and the oxygen ions towards the positive electrode (anode). This process demonstrates water’s ability to conduct electricity and undergo significant chemical transformations.
Beyond Electrolysis: Exploring Related Concepts
The exploration of water’s electrical properties extends beyond electrolysis, encompassing a multitude of related concepts and applications. Voltaic cells harness chemical reactions to generate electricity, while electroplating and electrorefining utilize electrolysis to deposit or remove metal ions from surfaces. Galvanic cells, on the other hand, rely on chemical reactions to produce electrical energy, forming the foundation for batteries, accumulators, and fuel cells.
Triboelectric Effect: Inducing Charges through Contact
The triboelectric effect unveils another intriguing aspect of water’s electrical nature. When water droplets come into contact with certain materials, such as glass or plastic, they can acquire a negative charge, due to the transfer of electrons between the surfaces. This phenomenon, which involves charging water through contact, has applications in detecting and generating static electricity.
Piezoelectricity: Generating Charges from Mechanical Stress
Piezoelectricity, a remarkable property of certain materials, allows water droplets to generate electrical charges when subjected to mechanical stress. This effect finds widespread use in technologies like ultrasound imaging, sonar, and microphone transducers. By converting mechanical energy into electrical signals, piezoelectricity provides valuable insights into various physical phenomena.
Photoelectric Effect: Charging Water with Light
The photoelectric effect, another fundamental concept in electrochemistry, enables water droplets to acquire a negative charge when exposed to certain wavelengths of light. This phenomenon underpins the operation of solar cells, photodiodes, and photomultipliers, devices that convert light energy into electrical energy. The photoelectric effect highlights the diverse ways in which water can interact with electromagnetic radiation.
The journey through the electrical properties of water reveals a world of versatile electrochemistry, where various processes and phenomena converge to manipulate water’s electrical charge. From electrolysis to the photoelectric effect, water’s ability to conduct electricity, undergo chemical transformations, and generate charges has found widespread applications in science, technology, and industry. This exploration showcases the hidden depths of this seemingly simple molecule, underscoring its significance as a key player in numerous electrical processes.
Electrolysis: Unveiling the Secrets of Water’s Split Personality
Water, often perceived as a simple, neutral substance, holds a hidden secret: it can be decomposed into its constituent elements—hydrogen and oxygen. This fascinating transformation is made possible through the power of electrolysis, a process that utilizes electricity to unlock the hidden potential of water.
Electrolysis, derived from the Greek words “electro” (electricity) and “lysis” (decomposition), is a scientific marvel that harnesses the transformative power of electricity. When an electric current is passed through pure water, it acts as a catalyst, breaking down the strong chemical bonds that hold water molecules together. This process results in the liberation of hydrogen and oxygen gas, which can be collected separately.
The electrolysis of water is a fascinating phenomenon with a long history. In the early 19th century, English scientist Sir Humphry Davy conducted groundbreaking experiments that demonstrated the potential of electrolysis. His experiments paved the way for further advancements in the field, eventually leading to the development of practical applications such as fuel cells and hydrogen-powered vehicles.
How Electrolysis Works
The process of electrolysis involves submerging two electrodes (usually made of inert materials such as platinum or carbon) in pure water. When an electric current is passed through the electrodes, it creates an electrical gradient in the water. This gradient drives the positively charged hydrogen ions (H+) towards the negatively charged cathode, where they gain electrons and transform into hydrogen gas (H2). Simultaneously, the negatively charged hydroxide ions (OH-) are drawn to the positively charged anode, where they lose electrons and combine to form oxygen gas (O2), releasing water vapor as a byproduct.
The rate of electrolysis, which determines how quickly water decomposes, is influenced by several factors, including the strength of the electric current, the surface area of the electrodes, and the temperature of the water. By carefully controlling these parameters, scientists and engineers can optimize the electrolysis process to achieve desired results.
Applications of Electrolysis
Electrolysis finds numerous applications in various scientific and industrial fields. One of the most prominent uses of electrolysis is in the production of hydrogen fuel. Hydrogen is a clean-burning fuel that emits only water vapor, making it a promising alternative to fossil fuels. Electrolysis provides a sustainable and efficient way to generate hydrogen from renewable energy sources such as solar and wind power.
Another important application of electrolysis is in the electroplating industry. Electroplating is a process by which a thin layer of metal is deposited on the surface of another metal. This technique is used to enhance corrosion resistance, improve surface properties, and create decorative finishes. Electrolysis is essential in the electroplating process, as it provides the controlled deposition of metal ions onto the desired surface.
In the medical field, electrolysis is used for electrocautery, a technique that involves using an electric current to heat tissue and seal blood vessels during surgical procedures. Additionally, electrolysis is employed in water purification systems, where it removes impurities and undesirable substances from water, making it safe for drinking or industrial use.
Electrolysis, the process of decomposing water using electricity, is a versatile and powerful tool with a wide range of applications. From the production of hydrogen fuel to the electroplating of metals, electrolysis plays a vital role in various scientific and industrial fields. Understanding the principles of electrolysis unlocks the potential to harness the hidden power of water, leading to innovative solutions for energy, environmental, and medical challenges.
Related Concepts: Voltaic Cells, Electroplating, and Electrorefining
Electricity is not just about powering our homes and gadgets; it also plays a crucial role in the field of electrochemistry, the study of the relationship between electricity and chemical reactions. And water, despite its seemingly neutral nature, can be manipulated with electricity in fascinating ways.
One such application is the voltaic cell, a device that converts chemical energy into electrical energy. It’s like a portable power plant that uses the electrochemical reaction between two different metals immersed in an electrolyte solution (water with dissolved salts). The key here is that the metals have different tendencies to donate or accept electrons, creating a flow of electrons and, thus, electricity.
Another fascinating application is electroplating. This technique uses electricity to coat a conductive object with a thin layer of another metal. In this process, the object to be coated is immersed in an electrolyte solution containing ions of the desired metal. When electricity is passed through the solution, the metal ions are deposited on the object’s surface, creating a durable, corrosion-resistant coating.
Finally, we have electrorefining. Similar to electroplating, electrorefining involves using electricity to purify metals. In this case, the impure metal is immersed in an electrolyte solution containing ions of the same metal. Electricity causes the impurities to separate from the metal, resulting in a purer form of the metal.
So, next time you think of water as just a neutral liquid, remember its hidden potential to dance to the tune of electricity. From generating electricity to purifying metals, the versatility of electrochemistry in water charging is truly remarkable!
Galvanic Cells: Generating Electricity from Chemical Reactions
Imagine a tiny, silent power source hidden within the depths of a simple chemical reaction. Welcome to the realm of galvanic cells, where electricity is born from the dance of ions.
A galvanic cell, also known as a voltaic cell, consists of two metal electrodes immersed in an electrolyte solution. When these electrodes are connected to an external circuit, a flow of electrons is triggered, creating an electrical current.
The secret to this electrochemical marvel lies in the chemical reactions occurring within the cell. Inside, one electrode, called the anode, undergoes an oxidation reaction, losing electrons. These electrons travel through the external circuit to the other electrode, known as the cathode, where they participate in a reduction reaction, gaining electrons.
The flow of electrons through the circuit generates an electrical current. The strength of this current depends on factors such as the difference in reactivity of the two electrodes and the concentration of the electrolyte solution.
Galvanic cells have been powering our world for centuries. They are found in devices like batteries, accumulators, and fuel cells.
- Batteries convert chemical energy into electrical energy by using up the reactants in the cell.
- Accumulators, such as rechargeable batteries, can be reused by reversing the chemical reactions.
- Fuel cells generate electricity from continuous reactions of fuels like hydrogen and oxygen.
The applications of galvanic cells are endless, from powering our smartphones to generating electricity in remote areas. Their versatility and efficiency have made them an indispensable tool in the realm of energy production.
The Power of Galvanic Cells: Unlocking Electricity from Chemical Reactions
In the world of electrochemistry, galvanic cells reign supreme. These devices, also known as voltaic cells, possess the remarkable ability to convert chemical energy into electrical energy. They’re the driving force behind a wide range of technologies, from everyday batteries to futuristic fuel cells.
Batteries: Powering Our Portable Lives
Think of batteries as mini powerhouses that store electrical energy chemically. Inside a battery, two electrodes made of different materials are immersed in an electrolyte solution. When connected, the chemical reactions within the battery create an electrical current. This current flows through an external circuit, powering our phones, laptops, and countless other gadgets that keep us connected and entertained.
Accumulators: Recharging the Power Reserves
Accumulators, a special type of battery, have the unique ability to be recharged. They store chemical energy that can be converted into electrical energy when needed. Lead-acid accumulators, widely used in cars, are a classic example. Through repeated charging and discharging, these accumulators keep our vehicles running smoothly.
Fuel Cells: The Future of Clean Energy
Fuel cells take electrochemistry to new heights by generating electricity from the reaction of a fuel (such as hydrogen) with oxygen. Unlike batteries, fuel cells do not store energy themselves. Instead, they produce electricity as long as there is a continuous supply of fuel. Fuel cells hold immense promise for powering vehicles, homes, and even entire communities with clean and sustainable energy.
From powering our devices to propelling our vehicles, galvanic cells play a vital role in modern life. Their versatility and efficiency make them indispensable in numerous applications, ranging from portable electronics to the pursuit of renewable energy sources. Electrochemistry continues to unlock new possibilities, harnessing the power of chemical reactions to shape our technological future.
The Curious Case of Water’s Electrical Antics: Unraveling the Triboelectric Effect
Imagine a world where the seemingly mundane act of touching an object could bestow upon a liquid the ability to attract or repel other objects. Enter the triboelectric effect, a fascinating phenomenon that unveils the hidden electrical nature of water.
When two dissimilar materials are brought into contact, a transfer of electrons occurs, creating an imbalance of charges. This electrical separation is what drives the triboelectric effect. As the materials separate, one surface gains a positive charge while the other acquires a negative charge.
In the realm of water, the triboelectric effect becomes particularly intriguing. When water droplets interact with certain materials, such as glass or plastic, they can accumulate a negative charge. This is because water has a high affinity for electrons, attracting them from the surface it comes into contact with.
This negative charge on water droplets has remarkable consequences. It can cause them to repel each other, creating mesmerizing displays of suspended droplets in midair. It also explains why water can sometimes cling to surfaces or form droplets that appear to float.
The triboelectric effect has found numerous applications in science and technology. It is the driving force behind static electricity, the crackling phenomenon that can make your hair stand on end or cause clothes to cling together.
Electroscopes and Van de Graaff generators harness the triboelectric effect to detect and create static charges, respectively. Microphones and ultrasound imaging devices utilize the effect to convert mechanical vibrations into electrical signals, allowing us to hear sounds and visualize objects in the human body.
Understanding the triboelectric effect provides a glimpse into the hidden electrical world that exists around us. It reveals that even ordinary substances like water can carry an electrical charge, exhibiting unexpected behaviors that have shaped our technological landscape.
Understanding Water’s Electrical Versatility
Water, the elixir of life, is often perceived as a neutral substance. However, within its seemingly inert depths lies a wealth of electrical phenomena that unveil the versatile nature of this precious resource.
Static Electricity: The Dance of Charged Particles
Through the triboelectric effect, water can acquire an electrical charge. When certain materials, such as glass or plastic, rub against water, electrons transfer, creating a static charge. This phenomenon is responsible for the crackling sensation one experiences after removing a sweater or combing their hair.
Van de Graaff Generator: Harnessing Static’s Potential
The Van de Graaff generator leverages the triboelectric effect to generate static electricity. A belt continuously transfers charge from one roller to another, building up a towering potential difference. The result? A dome-shaped apparatus that crackles with energy and can even make your hair stand on end!
Electroscope: Detecting Nature’s Electrical Whispers
The electroscope is a delicate instrument that detects the presence of static electricity. A lightweight metal leaf hangs suspended, swaying in response to nearby electrical charges. Its movements reveal the invisible electrical forces that permeate our environment.
The Piezoelectric Effect: Harnessing Mechanical Energy to Charge Water
In the realm of electrochemistry, piezoelectricity emerges as a fascinating phenomenon that allows us to generate electrical charges from mechanical stress. This incredible property has opened up innovative avenues for charging water and manipulating its electrical properties.
At the heart of piezoelectricity lies the ability of certain materials, known as piezoelectrics, to convert mechanical energy into electrical energy. When subjected to mechanical forces, these materials undergo a deformation that causes an internal charge separation. This charge separation results in the accumulation of opposite charges on different surfaces of the material, creating an electrical potential.
In the context of water charging, piezoelectricity can be utilized to induce electrical charges on water droplets. As mechanical stress is applied to the water, the piezoelectric effect generates a potential difference between the water and the surrounding environment. This potential difference, in turn, drives the movement of electrons, resulting in the accumulation of a negative charge on the water droplets.
The piezoelectric effect has found practical applications in various technologies that harness mechanical vibrations or deformations to generate electricity. These include:
- Ultrasound imaging: Piezoelectric transducers are used to generate and detect ultrasound waves, allowing for non-invasive imaging of the human body.
- Sonar: Piezoelectric materials are employed in sonar systems to emit and receive sound waves, enabling underwater navigation and object detection.
- Microphones: Piezoelectric microphones convert sound waves into electrical signals, capturing audio for recording and communication devices.
By understanding the principles of piezoelectricity and its role in charging water, we gain deeper insights into the versatile applications of electrochemistry in manipulating the electrical properties of water. This knowledge paves the way for advancements in fields as diverse as water purification, microfluidics, and energy harvesting.
Piezoelectricity: The Power of Pressure
The piezoelectric effect is a fascinating phenomenon where certain materials generate electrical charges when subjected to mechanical stress. This unique property has found remarkable applications in various technologies, including ultrasound imaging, sonar, and microphones.
Ultrasound Imaging: A Journey into the Body
Ultrasound imaging, a widely used medical diagnostic tool, harnesses the piezoelectric effect to visualize internal organs and tissues. Piezoelectric transducers emit ultrasonic waves that travel through the body, reflecting off tissues and returning to the transducer. The time and intensity of these echoes provide detailed images, enabling doctors to diagnose and monitor a wide range of medical conditions.
Sonar: Mapping the Underwater World
Sonar, a cornerstone of marine navigation and exploration, also relies on piezoelectricity. Piezoelectric transducers emit sound waves into the water, which bounce off objects and return to the transducer. By analyzing the time and intensity of these echoes, sonar systems create detailed maps of the underwater environment, guiding ships, detecting submarines, and studying marine life.
Microphones: Capturing the Sound of the World
Microphones convert sound waves into electrical signals, and many rely on piezoelectric materials. When sound waves strike a piezoelectric diaphragm, it vibrates, generating electrical charges. These charges are then amplified and processed to produce audio signals, allowing us to record, listen to, and communicate with sound.
The piezoelectric effect exemplifies the versatility of electrochemistry in manipulating the electrical properties of water. From medical imaging to underwater exploration and the transduction of sound, piezoelectricity has revolutionized numerous fields. Its ability to convert mechanical energy into electrical energy and vice versa has paved the way for countless innovations and continues to shape the world we live in.
Photoelectric Effect: Charging Water with Light
A Tale of Electrons and Photons
In the realm of electrochemistry, the photoelectric effect stands as a remarkable phenomenon, providing a novel way to charge water droplets with light. This effect is a testament to the intricate interplay between light and matter, where photons, the fundamental units of light, carry energy capable of liberating electrons from certain materials.
When photons strike the surface of water droplets, their energy can be absorbed by the water molecules. If the photon’s energy exceeds the ionization energy of water (the energy required to remove an electron from a water molecule), an electron is ejected from the molecule. This process is known as the photoelectric effect.
The freed electron leaves behind a positively charged water molecule, creating an ion pair within the water droplet. This ion pair disrupts the droplet’s electrical neutrality, inducing a negative charge on the water droplet. The magnitude of the charge is dependent on the number of photoelectrons emitted and the intensity of the light.
This remarkable phenomenon has opened up new avenues for electrochemical manipulations of water. By harnessing the power of light, researchers and engineers can precisely control the electrical properties of water droplets, paving the way for innovative applications in various fields.
Solar Cells, Photodiodes, and Photomultipliers: The Power of Light in Water Charging
The photoelectric effect is a phenomenon where light interacts with matter, causing electrons to be emitted. This effect is harnessed in various devices to generate electricity or detect light:
Solar Cells: Solar cells, also known as photovoltaic cells, are devices that convert sunlight directly into electricity. They utilize the photoelectric effect to generate an electric current when exposed to light. Solar cells are commonly used in solar panels to harness renewable energy for power generation.
Photodiodes: Photodiodes are semiconductor devices that convert light into an electrical signal. They operate based on the photoelectric effect, where incident light creates electron-hole pairs in the semiconductor material, generating a photocurrent. Photodiodes are widely used in light detection applications, such as sensors in cameras, optical communication systems, and medical imaging.
Photomultipliers: Photomultipliers are highly sensitive light detectors that amplify extremely faint light signals. They utilize the photoelectric effect in a cascade of stages, where each stage multiplies the number of electrons released by incident light. Photomultipliers are employed in applications that require exceptional light sensitivity, such as astronomical observations, nuclear physics experiments, and medical imaging.
The photoelectric effect has revolutionized our ability to harness light energy and detect faint light signals. Solar cells provide us with sustainable and renewable energy sources, while photodiodes and photomultipliers enable advanced applications in various scientific and technological fields.