Understanding Threshold Frequency: A Key Concept In The Photoelectric Effect
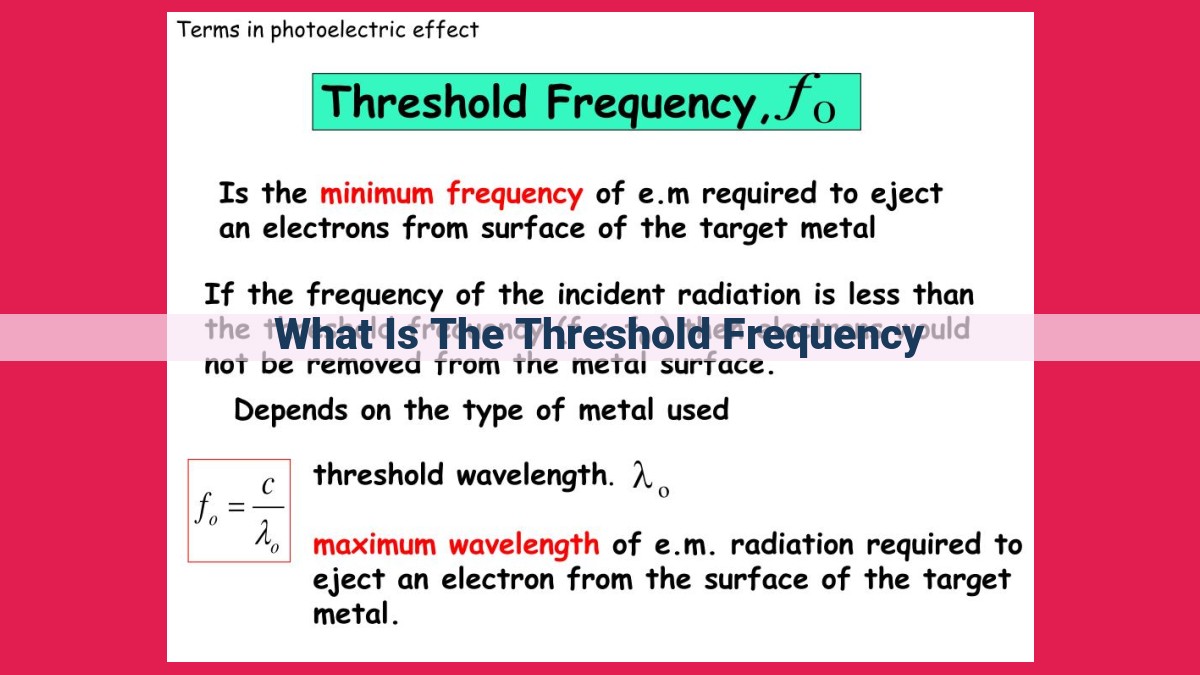
Threshold frequency is the minimum frequency of light required to eject electrons from a material’s surface via the photoelectric effect. When light with a frequency below the threshold frequency strikes the material, no electrons are emitted. Above the threshold frequency, electrons absorb energy from the light and are emitted with kinetic energy proportional to the difference between the light’s energy and the material’s work function. This relationship reveals the quantum nature of light and the discrete nature of energy absorption by electrons.
In the realm of physics, understanding the interaction of light with matter is crucial. One key concept that has significantly shaped this understanding is threshold frequency. This phenomenon holds profound significance in comprehending the photoelectric effect, a groundbreaking discovery that earned Albert Einstein the Nobel Prize in Physics.
Defining Threshold Frequency
Threshold frequency is a pivotal concept that describes the minimum frequency of electromagnetic radiation required to trigger the photoelectric effect in a given material. This threshold frequency varies depending on the material’s intrinsic properties. When light with a frequency below the threshold frequency strikes a material, the electrons remain bound to the material. However, when the light’s frequency surpasses the threshold frequency, something extraordinary happens.
Significance in the Photoelectric Effect
The threshold frequency plays a crucial role in initiating the photoelectric effect. When light with a frequency greater than the threshold frequency strikes a material, it imparts enough energy to the electrons to overcome the material’s work function – the binding energy holding the electrons in place. This energy transfer liberates the electrons, causing them to be emitted from the material’s surface.
The threshold frequency, therefore, determines the minimum energy required to initiate the photoelectric effect. This phenomenon unveils a fascinating insight into the quantized nature of light-matter interactions. By understanding threshold frequency, we gain a deeper comprehension of how light interacts with matter, facilitating a range of applications in fields such as photovoltaics, quantum computing, and many more.
Threshold Frequency and the Photoelectric Effect
In the realm of physics, a remarkable phenomenon known as the photoelectric effect has captivated scientists. At the heart of this effect lies a crucial concept called threshold frequency, which serves as a gateway to understanding the intricate dance between light and matter.
Threshold frequency, in essence, is the minimum frequency of light required to trigger the photoelectric effect in a given material. When light strikes a material, it imparts energy to its electrons. If the light’s frequency falls below the threshold frequency, this energy is not sufficient to overcome the material’s grip on the electrons.
However, when the light’s frequency reaches or exceeds the threshold frequency, a remarkable transformation occurs. The electrons absorb the light’s energy and are liberated from the material’s clutches. This ejection of electrons is the essence of the photoelectric effect.
The significance of threshold frequency lies in its ability to unravel the interplay between light and matter. It reveals that electrons have quantized energy levels and that the energy transfer between light and electrons occurs in discrete, quantized packets known as quanta.
Furthermore, threshold frequency provides a direct insight into a material’s electronic structure. The higher the threshold frequency, the stronger the material’s hold on its electrons, indicating a wider energy gap between the occupied and unoccupied energy levels. Conversely, a lower threshold frequency signifies a smaller energy gap and a greater susceptibility to electron emission.
Related Concepts: Photoelectric Threshold
The photoelectric threshold is a crucial concept closely intertwined with the threshold frequency. It marks the minimum frequency of light that can eject electrons from a material. When light with a frequency below the threshold frequency strikes a material, no electrons are emitted.
Imagine a scenario where you have a photon of light bouncing off a material. If the photon’s energy is below the material’s threshold, it’s like a weak knock at the door that doesn’t have enough force to open it. The electron remains peacefully inside the material.
However, if the photon’s energy exceeds the threshold, it’s like hitting the door with a battering ram. The energetic photon jolts the electron out of its cozy spot, causing it to escape. This is the essence of the photoelectric effect: light with sufficient energy can liberate electrons from materials.
Related Concepts: Work Function
The work function of a material is a crucial parameter that plays a significant role in determining the threshold frequency. It represents the minimum energy required to remove an electron from the surface of a material. As a material’s work function increases, so does its threshold frequency.
Mathematically, the work function (Φ) is directly proportional to the threshold frequency (f0):
Φ = h f0
where h is Plank’s constant.
This relationship highlights the inverse proportionality between work function and threshold frequency. A higher work function requires photons with a higher frequency to overcome the material’s resistance to electron emission, resulting in a higher threshold frequency. Conversely, a lower work function requires photons with a lower frequency to trigger the photoelectric effect, leading to a lower threshold frequency.
Understanding the work function of a material is essential for tailoring its response to specific light frequencies. For example, in photodetectors, materials with low work functions are preferred as they can detect low-frequency photons, while materials with high work functions are suitable for detecting high-frequency photons. This knowledge allows scientists and engineers to design devices that harness the interaction of light with matter based on the threshold frequency and work function of the materials involved.
Related Concepts: Quantum Energy
The captivating tale of quantum energy holds the key to unraveling the profound significance of threshold frequency in the intricate dance between light and matter. In this realm, energy is not a continuous flow but rather exists in discrete packets known as quanta. Each quantum possesses a specific magnitude of energy, and its presence within a system can profoundly alter its behavior.
When light interacts with matter, it carries with it a quantized energy content that depends on its wavelength. Threshold frequency marks the critical point at which the incoming light quanta, known as photons, possess sufficient energy to dislodge electrons from an atom. Below this frequency, photons lack the requisite energy to trigger the photoelectric effect, and no electrons are emitted.
The energy absorbed by electrons, upon exposure to the threshold frequency, plays a pivotal role in determining the velocity with which they are ejected from the material. This absorbed energy, in turn, is directly proportional to the work function of the material, a property that represents the minimum energy required to release an electron from its bound state.
In this context, the concept of quantum energy provides a fundamental framework for understanding the energy exchanges that occur during the photoelectric effect. It underscores the discrete nature of energy transfer between light and matter, ultimately illuminating the intricate interplay of these two realms.
Related Concepts: Electron Affinity
The Journey of an Electron: Exploring Electron Affinity
While not directly related to threshold frequency, the concept of electron affinity adds further context to our understanding of the behavior of electrons in response to light. Electron affinity refers to the energy change that occurs when a neutral atom gains an electron to form a negative ion. This energy change, typically expressed in electron volts (eV), represents the attraction between the positively charged nucleus and the newly acquired electron.
Imagine an electron as a curious wanderer, eager to find a comfortable home. When an atom opens its arms to welcome this wanderer, the electron gladly settles in, releasing energy as it does so. This energy release is known as the electron affinity.
Relevance to Photoelectric Effect
Although electron affinity is not directly involved in the photoelectric effect, it provides insights into the energetic interactions between electrons and light. The concept highlights the fact that electrons are not passive particles but rather dynamic entities that respond to changes in their surroundings.
Understanding electron affinity helps us appreciate the complexities of electron behavior and the diverse ways in which energy is exchanged in atomic interactions. It reminds us that the world of electrons is a vibrant and multifaceted realm, where seemingly unrelated concepts are often interconnected in surprising ways.