Thermal Energy Vs. Temperature: Understanding Heat And Equilibrium
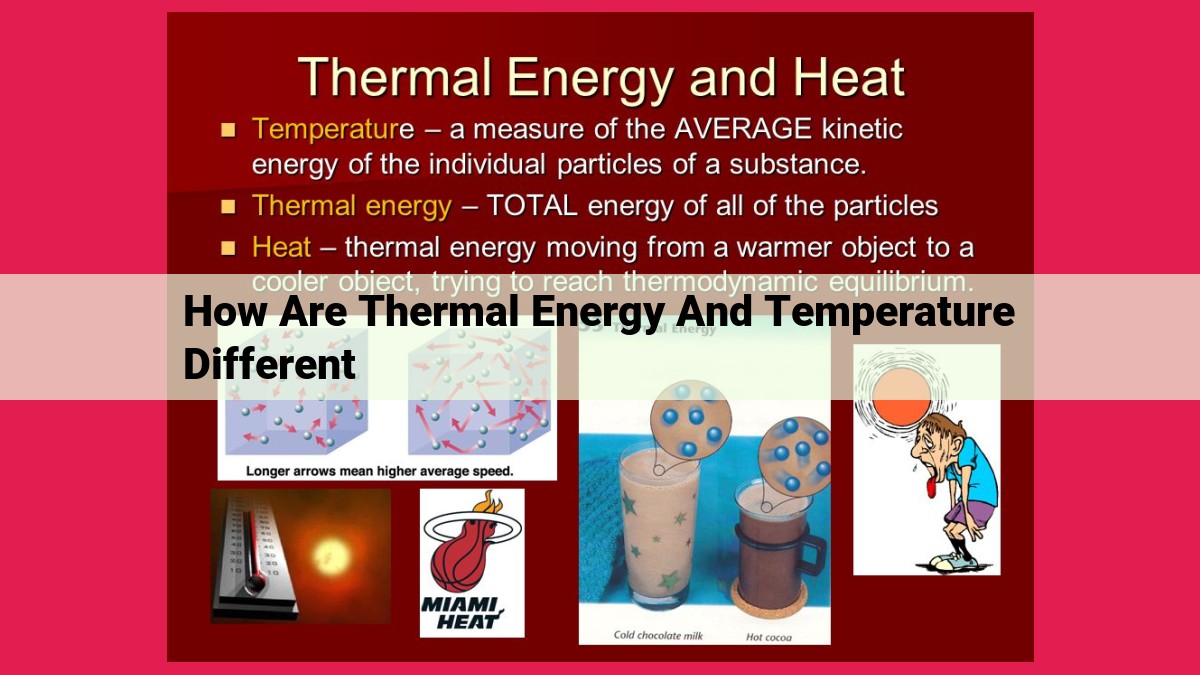
While thermal energy describes the total energy contained within a system due to the random motion of its particles, temperature measures the average kinetic energy of these particles and provides insight into the system’s heat intensity. In essence, thermal energy represents the amount of heat a system possesses, while temperature gauges its intensity. Thermal equilibrium occurs when systems with different temperatures exchange heat until they reach the same temperature, indicating an absence of heat flow.
Thermal Energy: The Essence of Heat
- Definition and explanation of thermal energy as a measure of molecular motion and potential energy.
Thermal Energy: The Essence of Heat
Embark on a thermal journey to unravel the enigmatic world of thermal energy, the invisible force that governs the temperature of our surroundings. This mysterious phenomenon, hidden within the microscopic realms of matter, holds the key to understanding how objects gain, lose, and exchange heat.
Thermal energy, an intrinsic property of matter, originates from the unceasing motion and vibrational energy of molecules. It is a quantitative measure of the kinetic and potential energy stored within these tiny building blocks. Imagine a bustling city teeming with inhabitants, each brimming with energy; thermal energy is the collective manifestation of this ceaseless activity on a molecular scale.
The greater the thermal energy possessed by a substance, the hotter it feels to the touch. This is because higher thermal energy corresponds to faster molecular motion and more intense vibrations, resulting in a sensation of warmth. Conversely, a substance with lower thermal energy feels cooler, as its molecules move more slowly and vibrate less vigorously.
The concept of thermal energy is essential for comprehending the fundamental nature of heat and temperature. As we delve deeper into this captivating subject, we will unravel the mysteries of thermal equilibrium, the enigmatic flow of heat, and the remarkable ability of materials to withstand temperature changes. Stay tuned for an enlightening exploration of thermal energy, the hidden force that shapes our thermal experiences.
Temperature: A Thermometer for Thermal Energy
Imagine a bustling city street during a sweltering summer day. The air is thick with heat, and the concrete beneath your feet radiates warmth. In such an environment, you can feel the thermal energy emanating from your surroundings. But what exactly is temperature, and how does it relate to this unseen energy?
Temperature: A Measure of Molecular Motion
Temperature is a measure of the average kinetic energy of the molecules in a substance. When molecules have high kinetic energy, they move faster and collide with each other more frequently. This increased molecular motion leads to a higher temperature.
Conversely, molecules with low kinetic energy move more slowly and collide less. This decreased molecular motion results in a lower temperature.
The Thermometer: A Window into Molecular Energy
A thermometer is a device that measures temperature by exploiting the relationship between temperature and molecular motion. As temperature increases, substances expand, and as temperature decreases, they contract.
A thermometer contains a liquid or gas that expands or contracts with changes in temperature. This expansion or contraction is measured and calibrated to provide a numerical value for the temperature.
Understanding Temperature and Thermal Energy
By understanding temperature, we can gain insights into the thermal energy of a substance. A high temperature indicates that its molecules possess a lot of kinetic energy, while a low temperature suggests that its molecules have less kinetic energy.
Temperature also plays a crucial role in heat transfer. Heat flows from substances with higher temperatures to substances with lower temperatures, as it represents the transfer of kinetic energy from high-energy molecules to low-energy molecules.
Thermal Equilibrium: The Harmony of Temperatures
In the realm of thermal physics, thermal equilibrium holds a pivotal place, akin to a celestial dance where temperatures synchronize and the flow of heat ceases to exist. Imagine a universe where objects, like celestial bodies, possess varying temperatures. Each object is a reservoir of thermal energy, its molecules bustling with motion and potential energy, manifesting as heat.
As these objects interact, a profound dance unfolds – heat exchange. Heat, the invisible yet potent force, flows from warmer bodies to cooler ones, like a celestial current. This ceaseless transfer continues until an equilibrium point is reached, a state of thermal harmony. At this celestial rendezvous, the temperatures of the objects become uniform, and the cosmic ballet of heat exchange comes to a halt.
Thermal equilibrium is not merely a static state of uniformity. It is a dynamic balance, where the temperature gradient that once drove heat flow has vanished. The molecules within each object, like synchronized dancers, now possess the same average kinetic energy, a measure of their thermal jostling. This synchronized motion reflects the equal distribution of thermal energy throughout the system.
Maintaining thermal equilibrium requires constant vigilance, as any perturbation can disrupt this delicate balance. A sudden influx of heat, like a starburst in the celestial tapestry, can upset the equilibrium, causing temperatures to diverge and reigniting the flow of heat. Yet, the system strives to re-establish its harmony, like a celestial orchestra adjusting to a maestro’s baton, until thermal equilibrium is once again achieved.
Understanding thermal equilibrium is crucial in diverse fields, from thermodynamics to astrophysics. It explains why a cup of hot coffee gradually cools to room temperature, as it exchanges heat with its surroundings, eventually reaching thermal equilibrium. It also underpins the functioning of stars, which maintain a delicate balance of fusion and radiation, ensuring the stability of our celestial neighborhood.
In the intricate symphony of the universe, thermal equilibrium plays a harmonious and essential role. It is a testament to the fundamental forces that shape our physical world, maintaining the delicate balance that allows life to thrive in its thermal embrace.
Heat: The Essence of Thermal Energy Exchange
In the realm of physics, heat emerges as a fascinating concept that governs the fascinating flow of thermal energy. This energy, inherent in every substance, represents the ceaseless motion and potential energy of its constituent molecules. As temperatures rise, so does the average kinetic energy of these molecules, reflecting a frantic dance of atomic motion.
When two substances of contrasting temperatures come into contact, a remarkable phenomenon occurs: heat, the lifeblood of thermal energy, begins to flow. This transference seeks to achieve thermal equilibrium, a harmonious state where temperatures equalize and the frantic molecular dance synchronizes. It’s as if the universe yearns for a balanced distribution of thermal energy.
Heat, like a restless wanderer, relentlessly travels from regions of higher temperature to those of lower temperature. This tireless flow is driven by a relentless pursuit of equilibrium, a state where molecules find solace in a comforting embrace of equal energy. The magnitude of this thermal energy transfer is dictated by the substance’s specific heat capacity, a measure of its willingness to absorb or release heat. Materials with higher specific heat capacities, like water, are like thermal sponges, readily soaking up energy without experiencing significant temperature changes. Metals, on the other hand, tend to be more thermally aloof, requiring less energy to spark noticeable temperature shifts.
Understanding the nature of heat is fundamental to unraveling a myriad of natural phenomena, from the gentle caress of a warm breeze to the fiery dance of a crackling flame. It’s a concept that shapes our world, from the design of energy-efficient homes to the development of innovative cooling systems.
Specific Heat Capacity: Understanding a Material’s Thermal Tolerance
What is Specific Heat Capacity?
Imagine you have a pot of hot water and a pot of cold water. When you add a spoon to the hot water, it feels hot to the touch. But when you add the same spoon to the cold water, it feels cold. This difference in temperature sensation is because the two materials have different specific heat capacities.
Specific heat capacity is a measure of a material’s ability to absorb or release thermal energy. It represents the amount of energy required to raise the temperature of one gram of a substance by one degree Celsius or Kelvin.
Significance of Specific Heat Capacity
Specific heat capacity is a crucial property that influences how a material behaves when heated or cooled. Materials with high specific heat capacities can absorb or release a large amount of energy without experiencing significant temperature changes. This makes them suitable for applications where temperature stability is essential, such as in heat exchangers, cooling systems, and building insulation.
Conversely, materials with low specific heat capacities experience rapid temperature changes when heated or cooled. They are often used in applications where rapid heating or cooling is desired, such as in cooking utensils, heat sinks, and medical devices.
Examples of Specific Heat Capacities
The specific heat capacity of water is exceptionally high (4.18 J/g°C). This means that it takes a lot of energy to raise the temperature of water, making it an excellent coolant and a stable thermal reservoir.
Metals, such as iron and aluminum, typically have low specific heat capacities (0.45 J/g°C and 0.90 J/g°C, respectively). This allows them to heat up and cool down quickly, making them suitable for cooking utensils and heat exchangers.
Specific heat capacity is a fundamental property that plays a vital role in understanding a material’s thermal behavior. By knowing the specific heat capacity of a material, engineers, scientists, and consumers can select the appropriate materials for their specific applications, ensuring optimal performance and energy efficiency.