The Nervous And Skeletal Systems: A Dynamic Duo For Movement And Homeostasis
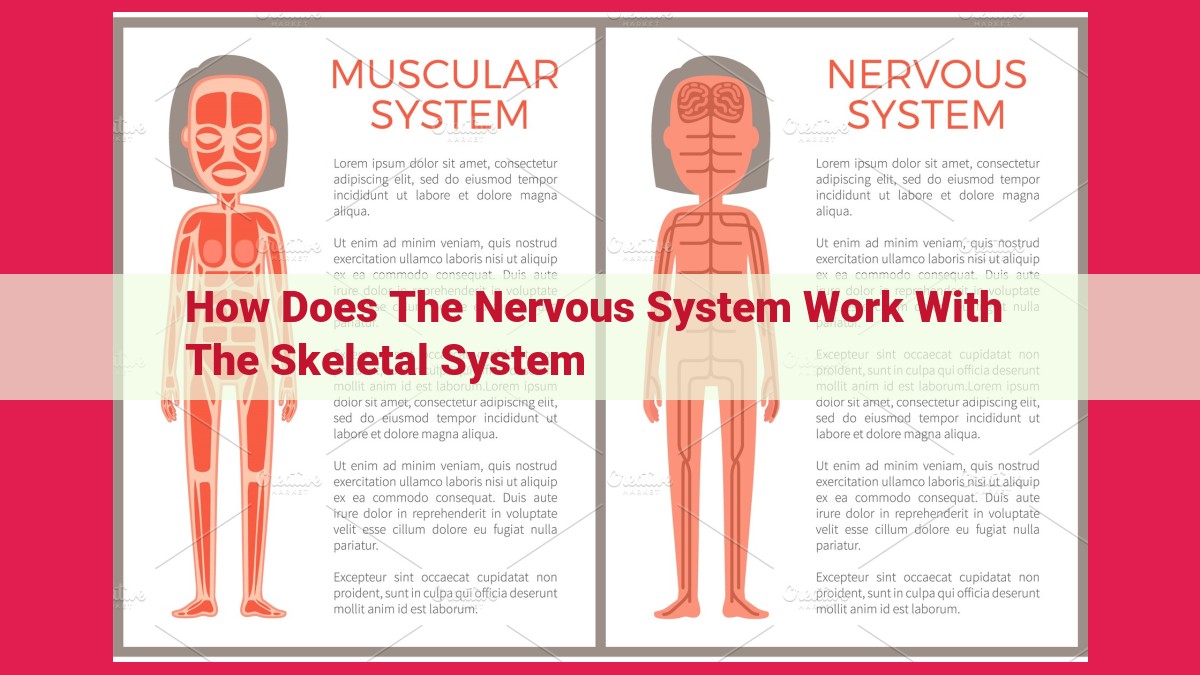
The nervous system and skeletal system collaborate to control body movements and maintain homeostasis. Sensory receptors detect stimuli and send signals to the central nervous system (brain and spinal cord), where motor neurons transmit commands to muscles. Reflex arcs facilitate rapid responses to stimuli. Voluntary and involuntary movements are controlled by different brain areas. Muscles contract through the sliding filament theory, facilitating movement. Joints act as levers, influencing range of motion. The cerebellum and vestibular system coordinate movements and maintain balance. Together, the nervous and skeletal systems regulate body functions through homeostasis, ensuring optimal conditions for movement and overall health.
Sensory Receptors and Motor Neurons: The Gatekeepers of Movement
Our bodies are constantly interacting with the world around us, gathering information through our senses to help us navigate and respond. These senses are made possible by sensory receptors, specialized cells that detect various stimuli, such as light, sound, heat, and touch. These receptors convert the stimuli into electrical signals that are then transmitted to the brain and spinal cord through the peripheral nervous system.
The brain and spinal cord, known collectively as the central nervous system (CNS), process the incoming sensory information and issue commands to the body accordingly. These commands are relayed to the muscles via motor neurons, which are the gatekeepers of movement. Motor neurons transmit signals from the CNS to specific muscles, causing them to contract or relax, enabling us to perform various movements.
Central Nervous System (CNS) and Peripheral Nervous System (PNS): A Symphony of Communication
The central nervous system (CNS) and peripheral nervous system (PNS) are two integral components of the nervous system that work in harmony to coordinate the body’s functions. The CNS, consisting of the brain and spinal cord, serves as the central processing unit, receiving and interpreting sensory information and sending commands to the body. The PNS, comprising cranial and spinal nerves, acts as a network of messengers, transmitting signals between the CNS and the rest of the body.
The CNS is the command center of the nervous system. The brain, the most complex and fascinating organ in the human body, processes sensory input, controls motor functions, and governs our thoughts, emotions, and memories. The spinal cord, a long, cylindrical structure, relays sensory and motor signals between the brain and the body’s peripheral regions.
The PNS, on the other hand, acts as the body’s communication network. Cranial nerves arise from the brain and innervate the head and neck regions, while spinal nerves emerge from the spinal cord and supply the trunk and limbs. These nerves transmit sensory information, such as touch, temperature, and pain, to the CNS and carry motor commands from the CNS to muscles and glands.
Together, the CNS and PNS form an intricate symphony of communication. Sensory receptors detect changes in the environment and send signals to the CNS, which processes this information and generates appropriate responses. Motor neurons then carry these responses from the CNS to muscles, causing them to contract and generating movement.
This constant exchange of information between the CNS and PNS enables the body to respond swiftly and effectively to both internal and external stimuli. It allows us to experience the world around us, control our movements, and maintain a stable internal environment—a testament to the remarkable interdependence of these two systems.
Reflex Arcs: Lightning-Fast Responses to Stimuli
In the realm of our bodies, rapid and involuntary responses to stimuli are crucial for our survival and well-being. These responses are orchestrated by a remarkable mechanism known as reflex arcs. Imagine a scenario where you accidentally touch a hot stove. In a flash, your hand withdraws without any conscious thought. This lightning-fast reaction is a testament to the efficiency of our reflex arcs.
A reflex arc is a specialized neural pathway that enables a rapid and involuntary response to a stimulus. It involves three key components: sensory receptors, motor neurons, and the central nervous system (CNS). Sensory receptors, located in various parts of the body, are responsible for detecting stimuli, such as heat, pain, or touch.
When a stimulus is detected, the sensory receptors convert it into an electrical signal. This signal is then sent along sensory neurons to the CNS, which consists of the brain and spinal cord. Within the CNS, the signal is processed and an appropriate response is determined. For example, in the case of touching a hot stove, the CNS sends a signal back to the hand via motor neurons, instructing the muscles to withdraw it.
Synapses, the junctions where neurons communicate, play a crucial role in reflex arcs. Neurotransmitters, chemical messengers, are released at synapses, facilitating the transfer of signals between neurons. This rapid and efficient communication allows for involuntary responses that protect us from harm and maintain our homeostasis.
Reflex arcs are essential for our survival, from the blink reflex that protects our eyes to the knee-jerk reflex that tests spinal cord function. They form the foundation of many of our bodily functions, ensuring our safety and well-being without the need for conscious thought.
Voluntary and Involuntary Movements: Control and Coordination
Our bodies are intricate symphony of motion, ranging from the graceful ballet of ballet dancers to the effortless beating of our hearts. These movements are powered by our nervous system and skeletal system, orchestrated by a complex interplay of voluntary and involuntary actions.
Voluntary Movements
Consciously controlled, requiring conscious thought and effort.
*Initiated by the *cerebral cortex, the ‘thinking’ part of the brain.
*Involve planning, decision-making, and execution.
*Examples: walking, talking, writing, playing sports.
Involuntary Movements
Automatic, not under conscious control.
*Triggered by external stimuli or internal signals.
*Controlled by the *brainstem, spinal cord, and autonomic nervous system.
*Examples: breathing, digestion, heart rate, reflexes.
Cerebellum and Basal Ganglia
These brain regions play pivotal roles in voluntary movement control:
- Cerebellum: Coordinates muscle activity, ensuring smooth, precise movements.
- Basal ganglia: Initiates voluntary movements, prevents unwanted movements.
Homeostasis and Control
The nervous system and skeletal system work together to maintain homeostasis, keeping our bodies in a stable internal environment.
The delicate dance of voluntary and involuntary movements is a testament to the marvels of the human body. From the effortless beating of our hearts to the intricate movements of a pianist, our bodies are a symphony of motion, carefully orchestrated by our nervous and skeletal systems.
Muscle Contraction: The Microscopic Symphony of Movement
Imagine your body as a complex orchestra, with muscles as the instruments that produce the symphony of movement. These intricate structures are the workhorses behind every twitch, stride, and graceful leap. But how do they perform this remarkable feat? The answer lies in the molecular ballet known as muscle contraction.
The Sliding Filament Theory
At the heart of muscle contraction lies the sliding filament theory. This theory proposes that muscles consist of two types of protein filaments: actin and myosin. These filaments are arranged in overlapping arrays called sarcomeres.
When a nerve impulse reaches a muscle, calcium ions flood into the cell. These ions bind to a protein called troponin, which triggers a conformational change in the actin filaments. This change exposes binding sites for myosin heads.
The Dance of Actin and Myosin
With the binding sites exposed, myosin heads attach to the actin filaments like tiny dancers. These heads possess an ATPase enzyme that hydrolyzes ATP (adenosine triphosphate), releasing energy that powers the contraction.
As myosin heads bind and hydrolyze ATP, they pull the actin filaments past each other. This sliding action causes the sarcomeres to shorten and the muscle to contract. The cycle repeats itself, with myosin heads detaching and reattaching to actin filaments to maintain the contraction.
The Role of Sarcomeres and Calcium
Sarcomeres are the basic units of muscle contraction. They contain the overlapping actin and myosin filaments and provide the structural framework for muscle movement.
Calcium ions play a crucial role in initiating and maintaining muscle contraction. Calcium influx triggers the conformational change in actin filaments, allowing for the binding of myosin heads. When calcium levels decrease, the contraction process ceases and the muscle relaxes.
Involuntary Movements: Smooth and Automatic
Muscle contraction is not limited to voluntary movements like walking and talking. It also powers involuntary functions such as breathing, digestion, and heart contractions. These involuntary movements are controlled by the autonomic nervous system, which operates without conscious thought.
Homeostasis: Orchestrating Internal Balance
The nervous and skeletal systems work in concert to maintain homeostasis, the stable internal environment essential for life. The nervous system monitors physiological parameters such as blood pressure and temperature, and triggers muscle contractions to adjust these parameters as needed. For example, when body temperature drops, the nervous system activates contractions in skeletal muscles to generate heat and maintain体温.
Joint Mechanics: Levers and Range of Motion
The human body is a masterpiece of biomechanics, where every movement is a testament to the intricate coordination between our nervous system and musculoskeletal system. Joints, the points where our bones meet, play a crucial role in facilitating this movement, acting as levers that amplify force and provide a wide range of motion.
Think of a joint as a fulcrum, like the one you might use to pry open a stubborn lid. When you apply force to one end of the lever, the other end moves in the opposite direction. In the case of our joints, the force is generated by our muscles, which contract and pull on the bones attached to the joint.
The type of joint determines the kind of movement it allows. Hinge joints, like those in our knees and elbows, provide up-and-down motion or flexion and extension. Ball-and-socket joints, such as the hip and shoulder, allow for a wider range of movement, including rotation and abduction (moving away from the body).
The range of motion at a joint is influenced by several factors. Joint structure, for example, determines how far the bones can move without dislocating. The strength of the muscles that cross the joint also plays a role, as stronger muscles can generate greater force. Finally, flexibility, which refers to the ability of muscles and tendons to stretch, contributes to the extent of joint movement.
Understanding joint mechanics is essential for maximizing performance and preventing injuries. Proper warm-ups can increase flexibility and reduce the risk of strain, while strengthening exercises can improve muscle power and enhance joint stability. By optimizing these factors, we can unlock our full potential for movement and enjoy an active and healthy lifestyle.
Coordination and Balance: Smooth and Steady Movements
In the ballet theatre, the ballerina gracefully pirouettes, her movements fluid and in perfect equilibrium. In the bustling city streets, a pedestrian confidently navigates the crowd without losing their footing. Behind these seemingly effortless actions lies a remarkable symphony of coordination and balance, orchestrated by the nervous system and skeletal system.
At the helm of this intricate choreography is the cerebellum, perched at the back of the skull. This “little brain” plays a pivotal role in coordinating muscle actions, ensuring that movements are smooth, precise, and timely. It constantly receives sensory information from various sources, including receptors in the inner ear and muscles, and uses this input to adjust muscle activity.
The inner ear houses a delicate structure called the vestibular system. This system contains fluid-filled canals and tiny hair cells that sense changes in head position and movement. It sends this information to the cerebellum, which helps us maintain balance and spatial orientation.
The muscles also play a crucial role in coordination and balance. Stretch receptors embedded within muscles provide information about muscle length and tension. This feedback allows the cerebellum to fine-tune muscle movements, preventing overextension or instability.
When these systems work in harmony, we achieve the remarkable ability to execute complex movements with precision and grace. The ballerina’s pirouette becomes a testament to the intricate coordination between her nervous and skeletal systems. The pedestrian, seemingly oblivious to the surrounding chaos, navigates the bustling streets with confidence, thanks to the vestibular system’s unwavering balance.
It is in these moments of effortless movement and perfect equilibrium that we appreciate the incredible orchestration of our body systems. The coordination of the nervous system and the balance provided by the skeletal system allow us to navigate our environment with poise, confidence, and grace.
Homeostasis: Maintaining a Stable Internal Environment
In the symphony of biological processes that sustain our existence, the nervous system and skeletal system act as harmonious partners, ensuring the delicate balance that we call homeostasis. Homeostasis, the body’s ability to maintain a stable internal environment despite external fluctuations, is crucial for our well-being.
The nervous system, the command center of the body, continuously monitors internal and external conditions, using sensory receptors to gather information. This information is then relayed to the central nervous system (brain and spinal cord), which processes the signals and sends appropriate responses to the body’s muscles and glands.
The skeletal system, on the other hand, provides the structural framework that supports and protects our body. But beyond its mechanical prowess, the skeletal system also plays a vital role in homeostasis. Bones store minerals and produce blood cells, contributing to the overall regulation of the body’s internal environment.
Negative and positive feedback loops are fundamental to the body’s ability to maintain homeostasis. Negative feedback loops counteract deviations from a set point, while positive feedback loops amplify them. For instance, when the body temperature rises, the hypothalamus, a part of the brain, triggers sweating and dilation of blood vessels to cool down the body. This is an example of negative feedback, as the response opposes the initial change.
In contrast, positive feedback loops occur during childbirth. As the baby descends through the birth canal, the stretching of the cervix triggers the release of oxytocin, a hormone that stimulates contractions. These contractions, in turn, further stretch the cervix, creating a positive feedback loop that facilitates the delivery of the baby.
The interplay between the nervous system and skeletal system ensures that the body’s internal environment remains within a narrow, optimal range. From regulating body temperature to maintaining proper blood pH, the collaborative efforts of these systems are essential for our survival and well-being. Homeostasis is not a static state but rather a dynamic equilibrium, continuously adjusting and responding to the ever-changing demands of life.