The Elastic Limit: Understanding The Stress Threshold For Material Integrity
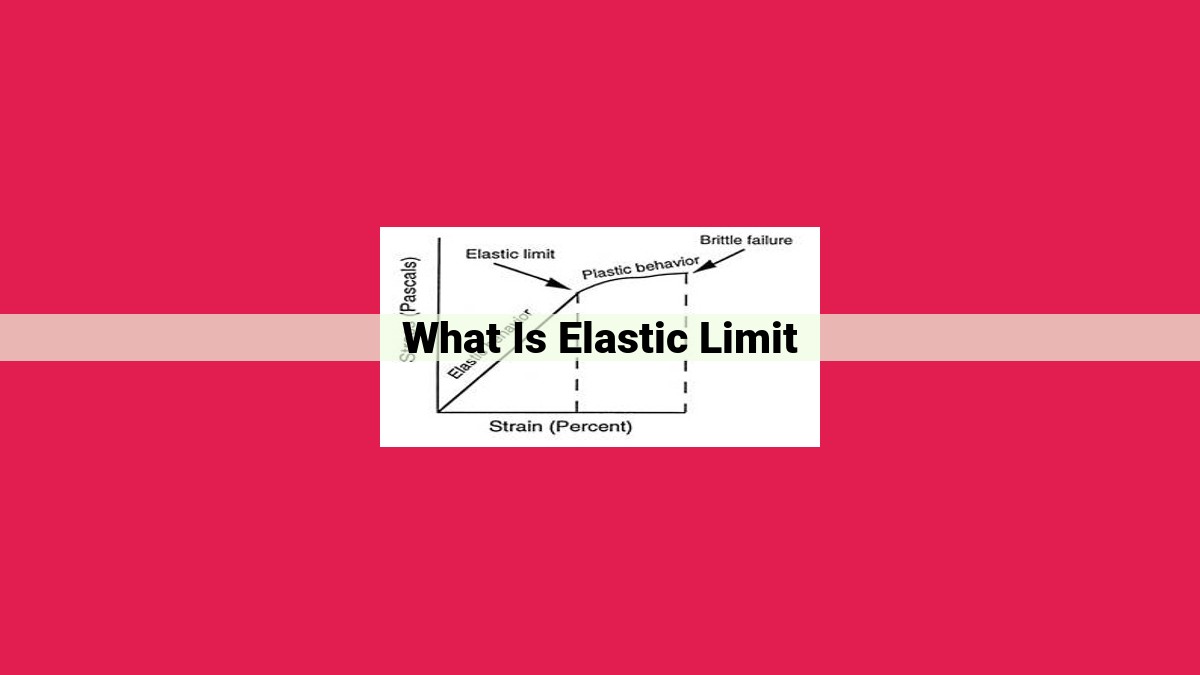
Elastic limit is the maximum stress a material can withstand without undergoing permanent deformation. Unlike tensile strength (ultimate stress) and yield strength, which represent failure points, elastic limit defines the boundary of recoverable elasticity. Factors like composition, temperature, and heat treatment influence this limit. Hooke’s Law relates stress and strain below the elastic limit, where the material behaves elastically. Engineers use it to select materials, design structures, and ensure safety. Testing methods determine the elastic limit for specific materials. Its implications are evident in real-world scenarios, affecting the performance and integrity of components and structures.
Unraveling the Elastic Limit: A Comprehensive Guide
In the realm of materials science, understanding the response of materials to external forces is crucial for ensuring the integrity and performance of structures. Among the diverse material properties, elastic limit stands out as a critical threshold that defines the limit of a material’s elastic behavior.
What is Elastic Limit?
The elastic limit is the maximum stress a material can withstand without undergoing permanent deformation. Beyond this limit, the material enters the plastic region, where deformation becomes irreversible. Unlike yield strength which represents the onset of plastic deformation, the elastic limit is not always clearly defined and can vary depending on the material and testing conditions.
Factors Affecting Elastic Limit
The elastic limit of a material is influenced by several factors, including:
- Composition: The chemical composition and microstructure of a material affect its interatomic bonding and, consequently, its elastic limit.
- Temperature: Elevated temperatures generally lower the elastic limit, making materials more susceptible to deformation.
- Heat Treatment: Heat treatment processes, such as annealing and quenching, can modify the material’s microstructure, altering its elastic limit.
Hooke’s Law and the Elastic Limit
Hooke’s Law establishes a linear relationship between stress and strain within the elastic limit. Stress is the force applied per unit area, while strain is the deformation experienced. According to Hooke’s Law, the ratio of stress to strain is a material property known as Young’s modulus. As stress approaches the elastic limit, the material’s ability to resist deformation becomes less linear.
Practical Applications of Elastic Limit
The elastic limit is a vital consideration in engineering applications, such as:
- Material Selection: Engineers select materials with an elastic limit that exceeds the expected loads and stresses in the application.
- Structural Design: Structures are designed to operate within the elastic limits of their components to prevent permanent deformation and failure.
- Safety Considerations: The elastic limit is used in safety assessments to determine the maximum load-bearing capacity of structures and components.
Testing for Elastic Limit
Determining the elastic limit of a material requires specialized testing equipment and protocols. Common testing methods include:
- Tensile Testing: A specimen is subjected to tensile load, with the elastic limit recorded as the point where deformation becomes non-linear.
- Compression Testing: Similar to tensile testing, but under compressive load.
- Bending Testing: A specimen is bent, and the elastic limit is identified as the point where permanent deformation occurs.
Elastic Limit in Real-World Scenarios
The elastic limit plays a critical role in the safety and integrity of structures in various real-world applications, such as:
- Bridges: The elastic limit of bridge components ensures they can withstand the weight and dynamic loading of vehicles without collapsing.
- Aircraft: The elastic limit of aircraft materials determines their ability to withstand aerodynamic forces and maintain structural integrity.
- Medical Implants: The elastic limit of implants must exceed the forces experienced in the body to prevent failure and ensure patient safety.
Common Misconceptions About Elastic Limit
It is important to dispel some common misconceptions about elastic limit:
- Elastic Limit is the Same as Yield Strength: While closely related, the elastic limit and yield strength are not identical. The elastic limit represents the onset of non-linear deformation, while the yield strength is the point where permanent deformation commences.
- All Materials Have a Clearly Defined Elastic Limit: The elastic limit can be somewhat ambiguous for certain materials, especially those with non-linear stress-strain behavior.
- Elastic Limit is Independent of Loading Conditions: The elastic limit can be influenced by factors such as loading rate, temperature, and environmental conditions.
**Factors Affecting Elastic Limit: Unraveling the Secrets of Material Elasticity**
The elastic limit, a crucial material property, defines the point beyond which a material will permanently deform under stress. Understanding the factors that influence this limit is essential for material selection and engineering design.
Composition: A Material’s Fingerprint
The chemical composition of a material is a key determinant of its elastic limit. Alloys, formed by combining different elements, often possess higher elastic limits than pure metals. For instance, steel’s high elastic limit arises from the presence of carbon and other elements that strengthen the atomic bonds.
Temperature: Heat’s Impact on Elasticity
Temperature also plays a significant role in elastic limit. As temperature increases, the atomic bonds within a material become weaker, reducing the material’s ability to resist deformation. This phenomenon explains why materials tend to have lower elastic limits at higher temperatures.
Heat Treatment: Tempering Strength and Flexibility
Heat treatment processes, such as annealing and tempering, can significantly alter a material’s elastic limit. Annealing involves heating and slowly cooling a material, which softens it and increases its elastic limit. Conversely, tempering involves heating and rapidly cooling a material, which hardens it and decreases its elastic limit.
Understanding the Elastic Limit Through the Lens of Hooke’s Law
In the realm of materials science, the elastic limit holds a significant position. It represents a critical point beyond which a material undergoes permanent deformation. Understanding this concept is essential for designing and using materials effectively.
One of the most fundamental relationships in mechanics is captured by Hooke’s Law. This law states that the stress applied to a material is directly proportional to its strain. In other words, as we apply more force to a material, it will stretch or compress by a predictable amount.
The elastic limit is the point on the stress-strain curve where this linear relationship breaks down. Beyond this point, the material begins to exhibit plastic deformation, meaning that it will not return to its original shape once the force is removed.
Hooke’s Law can be expressed mathematically as:
stress = modulus of elasticity * strain
Where:
- stress is the force per unit area applied to the material
- modulus of elasticity is a constant that represents the stiffness of the material
- strain is the deformation of the material per unit length
When the stress applied to a material exceeds its elastic limit, the material enters the plastic region. In this region, the material will continue to deform even if the stress is removed, resulting in permanent deformation.
The elastic limit is a crucial parameter for engineers and designers. It helps them ensure that materials used in structures and components will not fail under normal operating conditions. By understanding the relationship between stress, strain, and the elastic limit, engineers can make informed decisions about material selection and design to prevent catastrophic failures.
Practical Implications of Elastic Limit:
- Describe how the elastic limit is used in engineering applications, including material selection, structural design, and safety considerations.
How the Elastic Limit Shapes Engineering Applications
The elastic limit of a material, that delicate point where it transitions from elastic to plastic behavior, holds immense significance in the world of engineering. It’s a crucial parameter that guides engineers in making informed decisions about material selection, structural design, and safety considerations.
Material Selection:
The elastic limit plays a pivotal role in determining the suitability of a material for a specific application. Consider a suspension bridge, where the cables must bear tremendous loads yet remain elastic under extreme stress. Materials with a high elastic limit, such as high-strength steel, are the ideal choice for such critical components.
Structural Design:
Engineering structures, from skyscrapers to aircraft wings, must be designed to withstand predicted loads without exceeding their elastic limit. Engineers calculate the maximum stress that a structure will encounter and ensure that it remains below the elastic limit to prevent permanent deformation.
Safety Considerations:
The elastic limit is a safety benchmark. Suppose a structure is subjected to a load that exceeds its elastic limit. In that case, it will experience plastic deformation, compromising its structural integrity. Knowing the elastic limit allows engineers to set safety factors that ensure structures can handle unexpected loads without catastrophic failure.
Case Study: Aircraft Wing Design
The wings of an aircraft are a prime example of where the elastic limit is crucial. During flight, the wings experience bending stresses that must not exceed their elastic limit. If the wings were to permanently deform, the aircraft’s flight characteristics and safety would be severely compromised. Engineers carefully design wings using materials with a high elastic limit, ensuring they can withstand the aerodynamic forces without compromising structural stability.
Understanding the practical implications of the elastic limit is paramount in engineering. It empowers engineers to make informed choices about materials, design structures that are both strong and safe, and ultimately ensure the integrity of critical infrastructure.
Testing the Elastic Limit: Unraveling the Material’s Resilience
As we delve into the realm of materials science, understanding the elastic limit becomes paramount. It’s a crucial property that demarcates the threshold beyond which a material will irreversibly deform, yielding to the relentless forces acting upon it. To accurately determine this critical limit, a battery of tests are meticulously employed.
Tensile Testing: A Forceful Encounter
One widely used method is tensile testing. A specimen of the material is subjected to a gradually increasing tensile force, akin to a tug-of-war. The material’s stress-strain curve is meticulously charted, providing a graphical representation of its response to the applied force.
The Onset of Plasticity
As the force intensifies, the material’s elastic deformation reaches its peak. Beyond this elastic limit, the material exhibits plastic deformation, permanently altering its shape. A telltale yield point often marks this transition, discernible on the stress-strain curve as a departure from linearity.
Compression Testing: Exploring the opposite Force
Materials can also be tested under compressive force. Similar to tensile testing, a sample is subjected to a gradually increasing load, but this time in a compressive manner. The material’s elastic limit is determined by observing the point at which irreversible deformation occurs.
Other Methods: Exploring Diverse Approaches
In addition to these core methods, several other techniques can provide insights into a material’s elastic limit. Bend testing assesses a material’s flexibility under bending forces, while torsion testing gauges its resistance to twisting. Fatigue testing simulates repeated loading scenarios to uncover how materials withstand cyclic stresses over time.
Nondestructive testing methods offer valuable alternatives when preserving the integrity of the specimen is crucial. Techniques like ultrasonic testing and acoustic emission analysis can indirectly measure elastic properties without causing damage.
Knowing the elastic limit of a material enables engineers to make informed decisions. It guides material selection, ensuring components can withstand anticipated stresses without failing. It also informs structural design, preventing overstress and ensuring the safety and integrity of structures.
Elastic Limit in Real-World Scenarios
The Subtle Dance of Materials: Understanding Elastic Limit
Every material has a threshold, beyond which it deforms permanently. This threshold is known as the Elastic Limit, a critical property that dictates the safe operating zone of structures and components. Let’s explore some real-world examples that showcase the profound impact of elastic limit.
Bridges: A Delicate Balance
Imagine a majestic bridge spanning a mighty river, carrying countless vehicles and commuters daily. The elastic limit of the bridge’s structural materials determines its ability to withstand the constant strain of traffic and the relentless forces of nature. If the elastic limit is exceeded, the bridge’s stability could be compromised, potentially leading to catastrophic consequences.
Aircraft: The Importance of Integrity
In the realm of aviation, the elastic limit plays a crucial role in ensuring the safety and reliability of aircraft. The fuselage, wings, and other components must withstand the rigors of takeoff, flight, and landing. If the elastic limit is exceeded, the aircraft’s structural integrity can be compromised, putting lives at risk.
Building Resilience into Structures
From skyscrapers to humble homes, the elastic limit is paramount in ensuring the resilience of buildings against earthquakes, hurricanes, and other natural disasters. By designing structures that can withstand strains within the elastic limit, engineers safeguard the lives of occupants and protect property from catastrophic damage.
Common Misconceptions About Elastic Limit:
- Clarify any misconceptions or misunderstandings associated with elastic limit and its significance.
Common Misconceptions About Elastic Limit
When discussing the mechanical properties of materials, the elastic limit often sparks misunderstandings. To dispel these misconceptions and enhance your understanding, let’s clarify some key points:
-
Elastic Limit as a Precise Value: Contrary to popular belief, the elastic limit is not an exact value but rather a range of stresses where the material exhibits elastic behavior. This range can vary depending on the material’s composition and test conditions.
-
Elastic Recovery: Materials generally do not perfectly recover to their original shape after exceeding the elastic limit. Some permanent plastic deformation occurs, even at stresses below the yield strength.
-
Brittleness and Elastic Limit: Brittleness indicates a material’s tendency to fracture suddenly, not its elastic limit. Ductile materials that can undergo significant plastic deformation before fracture can still have a well-defined elastic limit.
-
Yielding and Plasticity: While yielding is often confused with the elastic limit, they differ subtly. Yielding marks the onset of plastic deformation, where the material irreversibly changes its shape. The elastic limit typically precedes yielding, but the two values may coincide for some materials.
-
Importance of Loading Conditions: The elastic limit can change with different types of loading. For example, a material’s tensile elastic limit may differ from its compressive elastic limit.