Temperature’s Impact On Cellular Respiration: Enzyme Activity, Adaptations, And Phenotypic Plasticity
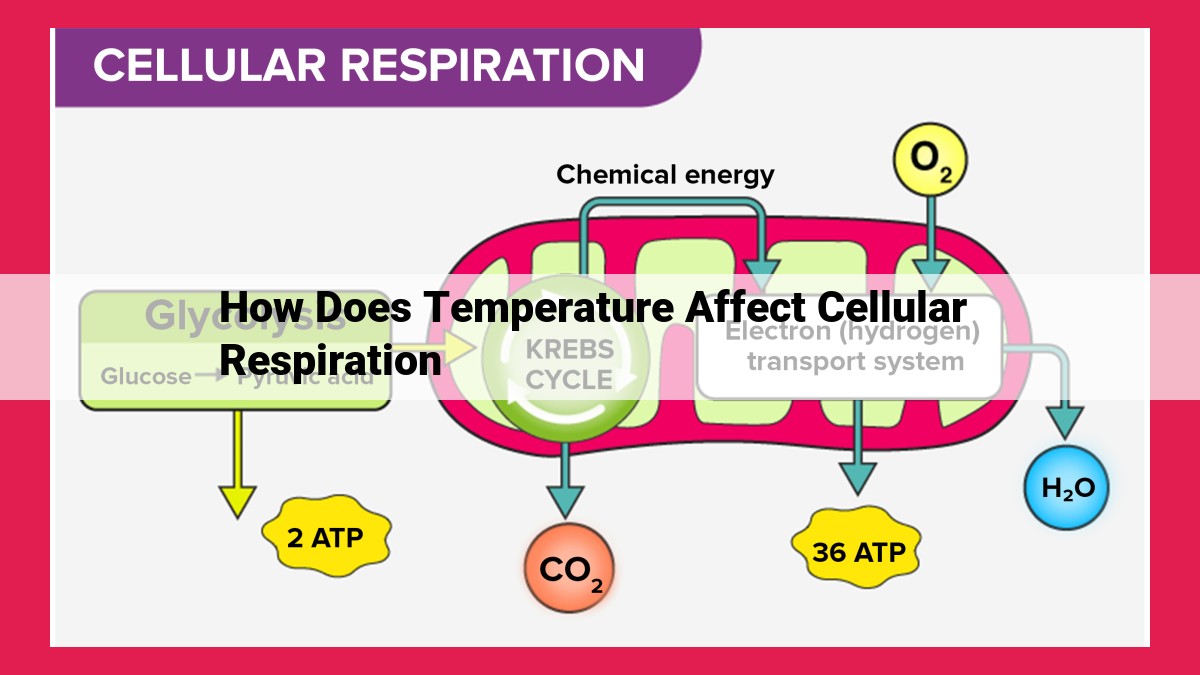
Temperature influences cellular respiration by affecting enzyme activity. The Q10 value describes the temperature effect on enzyme activity, with a higher Q10 indicating greater sensitivity to temperature changes. At high temperatures, enzymes denature, reducing their activity. Conversely, lower temperatures slow enzyme activity and alter substrate interactions. Organisms have evolved temperature-regulating mechanisms to maintain homeostasis, with adaptations to withstand extreme temperatures. Phenotypic plasticity allows for variations in temperature responses, ensuring survival in diverse environments.
Q10: The Enzyme Activity Temperature Regulator
- Definition of Q10 and its role in enzyme activity
- Arrhenius equation and its connection to Q10
Q10: The Enzyme Activity Temperature Regulator
Like a delicate dance, enzymes, the catalysts of life, delicately interact with molecules to choreograph the intricate symphony of chemical reactions within our cells. However, the rhythm of this dance can be disrupted by temperature fluctuations, demanding sophisticated adaptations for maintaining cellular harmony.
Enter Q10, a thermodynamic constant that measures the effect of temperature on enzyme activity. Q10 represents the fold increase in an enzyme’s catalytic rate with a 10-degree Celsius rise in temperature. This pivotal parameter reflects the intricate relationship between temperature and enzyme kinetics.
The Arrhenius equation, a fundamental cornerstone of chemical kinetics, provides a mathematical framework for understanding this relationship. It elegantly describes how the rate of chemical reactions increases exponentially with temperature, exhibiting a linear dependence on the inverse of temperature. The slope of this linear relationship, known as the activation energy (Ea), reflects the energy barrier that must be overcome for a reaction to occur.
By connecting the Arrhenius equation to Q10, we gain invaluable insights into the temperature dependence of enzyme activity. As Ea increases, so does the slope of the Arrhenius plot, indicating a greater sensitivity of the enzyme to temperature changes. Conversely, a lower Ea results in a shallower slope, suggesting a more resilient enzyme in the face of temperature variations.
Understanding Q10 and its connection to enzyme activity is critical for comprehending how organisms adapt to temperature fluctuations, a key aspect of life’s resilience.
Unveiling the Impact of High Temperature on Cellular Respiration: A Tale of Enzyme Inactivation
Cellular respiration, the powerhouse of our cells, relies on the precise orchestration of enzymes, each playing a vital role in the intricate process of energy production. However, when temperatures soar, these molecular maestros face an arduous challenge, jeopardizing the very lifeline of our cells.
At the heart of this heat-induced dilemma lies protein denaturation, a phenomenon where the delicate structure of enzymes unravels under extreme heat. Imagine an intricate puzzle, its pieces perfectly aligned to perform a specific task. As temperatures rise, these pieces lose their precise arrangement, compromising the enzyme’s ability to bind to its substrate, the reactant it must interact with to initiate the reaction.
This loss of binding affinity marks the beginning of enzyme inactivation. Like a key that no longer fits its lock, the enzyme can no longer perform its assigned task in cellular respiration. This disruption reverberates through the entire metabolic pathway, causing a cascade of consequences that can ultimately lead to cellular dysfunction.
Enzymes, the linchpins of cellular respiration, are particularly vulnerable to high temperatures. Their intricate molecular structures, fine-tuned for optimal activity at a specific temperature range, become unstable when exposed to excessive heat. This instability manifests as a decrease in enzyme activity, a crippling blow to the cell’s ability to generate energy.
In the face of such adversity, cells have evolved remarkable mechanisms to adapt to temperature fluctuations. Some organisms have heat-stable enzymes that can withstand higher temperatures without losing their functionality. Others possess the ability to synthesize new enzymes rapidly, compensating for those damaged by heat.
Understanding the impact of high temperature on cellular respiration not only sheds light on the fundamental processes that govern life but also has significant implications in fields such as medicine and biotechnology. By manipulating enzyme stability and cellular response to temperature, scientists can potentially develop novel therapies for diseases and improve the efficiency of industrial processes that rely on enzymes.
**The Chilling Impact: How Low Temperature Affects Cellular Respiration**
In the intricate dance of life, temperature plays a crucial role. When the mercury dips, cellular respiration, the energy-generating engine of our cells, experiences a chilling effect.
Slowed Enzyme Activity: A Symphony Disrupted
Enzymes, the maestro of chemical reactions, are sensitive to temperature fluctuations. As temperatures drop, enzyme activity takes a nosedive. This is because the lower kinetic energy of molecules at low temperatures hinders their ability to find each other and collide effectively. With fewer successful collisions, the rate of chemical reactions, including those essential for cellular respiration, slows considerably.
Alterations in Enzyme-Substrate Interactions: A Dance out of Sync
In addition to slowing enzyme activity, low temperatures can also alter enzyme-substrate interactions. Enzymes and substrates are like two partners in a dance, each with specific binding sites. As temperatures drop, the shapes of these binding sites can change, affecting how well the enzyme and substrate fit together. This altered affinity can lead to a breakdown in the dance, further impeding cellular respiration.
Consequences for Life: A Chain Reaction
Slowed enzyme activity and altered enzyme-substrate interactions have far-reaching consequences for cellular respiration. Energy production, the lifeblood of cells, dwindles. Metabolic processes, such as protein synthesis and DNA replication, sputter. If temperatures remain low for extended periods, cells may struggle to survive, disrupting the delicate balance of life.
Acclimation: A Tale of Survival
Faced with the cold, some organisms possess remarkable acclimation abilities. They can adapt to low temperatures by altering their enzyme composition, increasing their number of membrane-bound enzymes, or adjusting their substrate affinities. These adaptations allow them to maintain cellular respiration and thrive in cold environments.
Low temperatures have a chilling effect on cellular respiration. They slow enzyme activity, alter enzyme-substrate interactions, and impede essential metabolic processes. Understanding these temperature-dependent effects is crucial for unraveling the mysteries of life and the remarkable adaptations that allow organisms to survive in the face of environmental challenges.
Acclimation: Organisms’ Temperature Responses
Every living organism is subject to the unyielding force of temperature fluctuations. From the sweltering heat of deserts to the icy embrace of polar regions, organisms have evolved remarkable adaptations to cope with these environmental extremes.
Homeostasis and Temperature Regulation
All organisms strive to maintain a delicate balance within their internal environment, known as homeostasis. Temperature is a critical factor in this equation, as it directly affects enzyme activity and various physiological processes.
Adaptations for Coping with Extreme Temperatures
To survive extreme temperatures, organisms have developed an array of adaptations. Some heat-tolerant species produce heat-stable proteins and sweat to regulate body temperature. On the other hand, cold-tolerant organisms have adapted to withstand freezing temperatures by accumulating antifreeze proteins and reducing metabolic rates.
Phenotypic Plasticity and Variations in Temperature Responses
Even within a single species, individuals may exhibit phenotypic plasticity(or variations in traits) in response to temperature variations. For instance, some populations of the same species may develop different heat-tolerance thresholds based on their geographical location. This ability to adjust to different temperature ranges enhances their survival chances in diverse environments.
In conclusion, acclimation is a testament to the extraordinary resilience of life. By employing a myriad of strategies, organisms have mastered the art of adapting to a wide spectrum of temperatures, ensuring their survival and the continuation of life on Earth.