Sulfur: A Reactive Element With Six Valence Electrons For Chemical Bonding
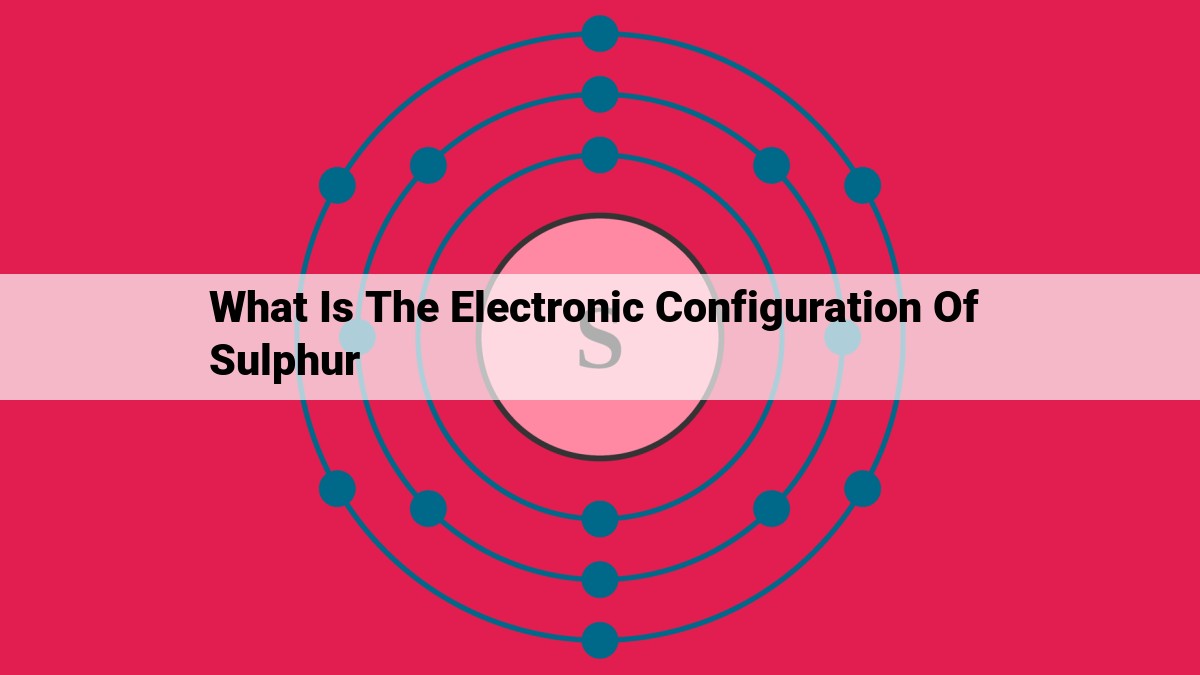
Sulfur, with an atomic number of 16, possesses an electronic configuration of [Ne]3s²3p⁴. This configuration consists of two electrons in the 3s subshell and four electrons in the 3p subshell, giving it a total of six valence electrons. The presence of six valence electrons makes sulfur highly reactive, as it can readily form bonds to achieve a stable octet configuration.
Electronic Configuration: Unlocking the Secrets of Chemical Behavior
Imagine yourself as a detective, embarking on an exciting quest to unravel the mysteries of the atomic world. Your first mission: to decipher the electronic configuration, the unique fingerprint that defines every element’s chemical behavior.
Electronic configuration refers to the arrangement of electrons, tiny particles that orbit around the nucleus of an atom. These electrons occupy specific energy levels, or shells, which determine their distance from the nucleus. Each shell can hold a maximum number of electrons, with the first shell holding up to 2 electrons, the second shell up to 8, and so on.
The outermost electrons, known as valence electrons, play a crucial role in chemical bonding. These electrons interact with electrons from other atoms, leading to the formation of chemical compounds with unique properties.
A key concept in understanding electronic configuration is the noble gas configuration. Noble gases, like helium and neon, are highly stable and unreactive elements because their outermost energy levels are completely filled with electrons. This arrangement makes them reluctant to participate in chemical reactions.
Atomic Number and Protons: The Foundation of Electronic Configuration
In the vast tapestry of the atomic realm, each element possesses a unique identity, defined by its atomic number. This number, a fundamental property, signifies the discerning characteristic that distinguishes one element from another. But how does the atomic number relate to the very building blocks of atoms — the protons?
Atomic Number: A Fingerprint of Identity
Imagine a colossal mansion with countless rooms, each housing a different number of occupants. The atomic number of an element acts like a unique address, identifying the exact mansion among the multitude. Just as the number of rooms determines the size of the mansion, the atomic number designates the number of protons residing within the atom’s nucleus.
Protons: The Positively Charged Core
These protons, positively charged particles, form the heart of an atom, nestled snugly in the nucleus alongside their neutral counterparts, neutrons. The protons’ presence not only defines the element but also governs the electronic configuration of the atom.
A Dance of Attraction and Repulsion
Like magnets with opposite poles, protons and electrons engage in a continuous waltz. The positive charge of protons exerts a relentless pull on the negatively charged electrons, drawing them towards the nucleus. However, electrons, like rebellious teenagers, also possess an inherent repulsion to one another. This delicate interplay between attraction and repulsion shapes the intricate dance of electrons around the nucleus.
The Significance of the Atomic Number
Understanding the atomic number and its relation to protons is crucial in unraveling the electronic configuration of an element. By knowing the atomic number, we can predict the number of protons and, subsequently, the number of electrons an atom will possess. This knowledge unlocks the gateway to comprehending the chemical properties and behavior of each element that graces our periodic table.
Electron Configuration
- Describe the concept of energy levels and orbitals.
- Discuss the number of electrons each energy level can hold.
Electron Configuration: Unraveling the Electronic Arrangement of Atoms
The electronic configuration of an atom describes the arrangement of its electrons across various energy levels. These energy levels are like imaginary shells surrounding the atomic nucleus, and each shell can hold a specific number of electrons.
The first energy level, closest to the nucleus, can accommodate up to two electrons. The second energy level can hold up to eight electrons, and the third energy level can accommodate up to 18 electrons. Each energy level is further divided into sublevels, which are designated as s, p, d, and f. Each sublevel has a specific shape and energy. For example, the s sublevel is spherical, while the p sublevel has a dumbbell shape.
The number of electrons an atom has determines its chemical behavior. Atoms with a full outermost energy level are chemically stable and are known as noble gases. Atoms with unfilled outermost energy levels are chemically reactive and tend to gain or lose electrons to achieve a stable configuration.
The Aufbau principle describes the order in which electrons fill the energy levels and sublevels. Electrons first occupy the lowest energy levels before moving to higher levels. Within each energy level, electrons fill the s sublevel first, followed by the p sublevel. The Pauli exclusion principle states that no two electrons within an atom can have the same set of four quantum numbers. The Hund’s rule states that electrons in the same sublevel tend to occupy different orbitals with parallel spins before pairing up in the same orbital.
Valence Electrons: The Key Players in Chemical Bonding
Valence electrons, the outermost electrons in an atom’s electron configuration, play a critical role in determining the atom’s chemical properties. They are the electrons that can participate in the formation of chemical bonds, the forces that hold atoms together to form molecules and compounds.
Understanding the Role of Valence Electrons
Imagine atoms as miniature solar systems, with the nucleus, consisting of protons and neutrons, at the center and the electrons orbiting around it. The outermost electrons, the valence electrons, reside in the highest energy level and are loosely bound to the nucleus. This loose attachment allows valence electrons to move freely, making them available for chemical bonding.
The Noble Gas Rule and Valence Electrons
The number of valence electrons an atom possesses is directly related to its chemical reactivity. Atoms with a full valence shell (eight valence electrons) are considered stable and have little tendency to react. These elements are known as noble gases.
Implication for Chemical Bonding
Elements with fewer than eight valence electrons seek to achieve a noble gas configuration by either gaining or losing electrons. For example, sodium (Na) has one valence electron, which it readily gives up to obtain a stable eight-electron configuration. On the other hand, chlorine (Cl) has seven valence electrons and tends to gain one electron to complete its shell.
When atoms lose or gain electrons, they become ions. Positive ions, or cations, are formed when atoms lose electrons, while negative ions, or anions, are formed when atoms gain electrons.
Valence electrons help determine the type of chemical bond that forms between atoms. In covalent bonds, electrons are shared between atoms, while in ionic bonds, electrons are transferred from one atom to another. The number and arrangement of valence electrons are crucial factors in predicting the behavior of atoms in chemical reactions.
Noble Gas Configuration: The Ultimate Electronic Stability
In the realm of chemistry, electronic configurations play a pivotal role in determining the properties and behavior of elements. These configurations describe the arrangement of electrons in the energy levels surrounding the nucleus of an atom.
Among the elements, noble gases stand out as beacons of stability. Their electron configurations hold a key to understanding why they are so unreactive.
Noble gases possess a complete set of electrons in their outermost energy level. This arrangement grants them an inert and stable state. The noble gas helium, for instance, has two electrons in its outermost energy level, giving it a stable and non-reactive nature.
The aufbau principle dictates that electrons fill orbitals in the order of increasing energy. However, in the case of noble gases, the outermost energy level is completely filled, and this creates a special configuration known as the octet rule. According to this rule, atoms with eight electrons in their outermost energy level exhibit exceptional stability.
The significance of the noble gas configuration lies in its impact on chemical bonding. Valence electrons, the electrons in the outermost energy level, participate in chemical reactions. However, noble gases have a full complement of valence electrons, making them reluctant to gain or lose electrons and thus resistant to forming chemical bonds.
In contrast, elements with incomplete valence electron configurations tend to be more reactive as they seek to achieve the stable configuration of a noble gas. For instance, sodium, with one valence electron, readily reacts with other elements to complete its outermost energy level and attain the stability of the noble gas neon.
Understanding the noble gas configuration is essential for comprehending the chemical behavior of elements. It explains the inertness of noble gases, the reactivity of elements with incomplete valence configurations, and the driving force behind chemical bonding.
Aufbau Principle: The Orbital Filling Rules
The Aufbau Principle is a fundamental concept in chemistry that dictates the way electrons fill atomic orbitals. It states that electrons will occupy the lowest energy orbitals available before moving on to higher energy levels. This principle helps us understand the electronic configurations of atoms and their chemical properties.
Imagine an atom as a miniature solar system, with the nucleus as the sun and the electrons orbiting around it like planets. Each orbit, or energy level, can hold a certain number of electrons, just like each planet can only hold a certain number of moons. The first energy level, closest to the nucleus, can hold two electrons. The second level can hold eight, and the third level can hold 18.
As electrons are added to an atom, they will always fill the lowest energy level first. For example, the first two electrons will occupy the 1s orbital, which is the lowest energy level. The next two electrons will go into the 2s orbital, and so on. Only when the lower energy levels are filled will electrons move on to higher energy levels.
The Aufbau Principle gives us a systematic way to predict the electron configurations of atoms. By following its rules, we can determine the number of electrons in each energy level and the arrangement of those electrons within the orbitals. This information is essential for understanding the chemical behavior of elements and the formation of molecules.
In summary, the Aufbau Principle is a fundamental principle that describes the filling of orbitals by electrons. It states that electrons will occupy the lowest energy orbitals available before moving on to higher energy levels. This principle helps us understand the electronic configurations of atoms and their chemical properties.
Hund’s Rule
- Explain the rule that determines the spin orientation of electrons in orbitals.
Hund’s Rule: Unraveling the Spin Dance of Electrons
Within the intricate world of atomic structure, electrons waltz around the nucleus in their designated energy levels. But how do these tiny particles decide their spin orientation, up or down? Enter Hund’s Rule, a guiding principle that governs the spin dance of electrons in orbitals.
Imagine a group of electron friends sharing an orbital, like a tiny dance floor. According to Hund’s Rule, they prefer to spread out their spins, like dancers trying to avoid bumping into each other. They’ll keep spinning apart, creating a state with the maximum possible spin multiplicity.
Why does this matter? It’s all about stability. By maximizing the spin multiplicity, the overall energy of the system is lowered, making it more favorable. It’s like a group of magnets that prefer to align their poles in the same direction, creating a stronger magnetic field.
In the case of sulfur, our protagonist atom, it has six valence electrons. According to Hund’s Rule, these electrons will occupy three separate orbitals with their spins aligned parallel to each other, creating a spin multiplicity of 3. This configuration contributes to sulfur’s unique chemical behavior and its ability to form diverse bonds with other elements.
So, there you have it, Hund’s Rule, a fundamental principle that shapes the spin dance of electrons in atoms. It provides a deeper understanding of atomic structure and lays the foundation for explaining the chemical properties of elements like sulfur and beyond.
Unveiling the Mystery of Electron Configurations: The Pauli Exclusion Principle
Embark on a thrilling odyssey into the enigmatic world of electron configurations, where electrons dance elegantly within an atom’s embrace. In this captivating blog, we unravel the captivating tale of the Pauli Exclusion Principle, a cornerstone of quantum physics that governs the intricate choreography of electrons, preventing them from occupying the same quantum space.
Picture an atomic landscape teeming with energetic electrons, each yearning for a place to reside. Like celestial dancers, they gracefully leap from one energy level to another, their movements dictated by the fundamental principles of physics. Electrons, like skilled performers, adhere to a strict code, the Pauli Exclusion Principle. This principle asserts that no two electrons within an atom can share the same set of quantum numbers. It’s as if each electron possesses a unique identity, a celestial fingerprint that sets it apart from its companions.
The Pauli Exclusion Principle eloquently explains why electrons don’t pile up in a single energy level like unruly partygoers. Instead, they distribute themselves across available energy levels, creating a harmonious symphony of orbitals, each occupied by a solitary electron. This elegant arrangement ensures that the energy levels remain stable and prevents unruly electron behavior.
Imagine a celestial ballet, where electrons twirl and leap with grace. The Pauli Exclusion Principle acts as an invisible choreographer, guiding their movements, ensuring they never collide or overlap. It’s a testament to the intrinsic order that underpins the very fabric of our universe.
Delving deeper into the implications of the Pauli Exclusion Principle, we discover its profound impact on the chemical properties of elements. It explains why atoms seek to acquire a stable electron configuration, often resembling that of noble gases, with their outermost energy level filled with electrons. This quest for stability drives the fascinating realm of chemical reactions, where atoms interact to achieve their desired electron configurations.
So, as we conclude our journey into the world of electron configurations, let us marvel at the elegance and precision of the Pauli Exclusion Principle. It’s a principle that shapes the very heart of matter, ensuring the harmonious dance of electrons and the stability of our universe.
Electronic Configuration of Sulfur: Unveiling the Secrets of a Vital Non-Metal
In the vast realm of chemistry, understanding the electronic configuration of elements holds the key to unraveling their properties and behavior. Electronic configuration refers to the arrangement of electrons within an atom’s energy levels, providing vital insights into its chemical nature.
Sulfur’s Atomic Identity
Every atom is characterized by its atomic number, which represents the number of protons in its nucleus. Protons, along with neutrons, define an element’s identity. Sulfur, with an atomic number of 16, belongs to the non-metallic family.
Unveiling Energy Levels and Orbitals
Electrons reside within energy levels, also known as orbitals. Each energy level can accommodate a specific number of electrons, with lower levels closer to the nucleus. Orbitals, the regions where electrons are most likely to be found, come in different shapes.
Valence Electrons: The Key to Chemical Bonding
The outermost energy level, known as the valence shell, contains valence electrons. These electrons play a crucial role in chemical bonding, determining an element’s reactivity and ability to interact with others.
Noble Gas Configuration: A Stable Haven
Noble gases, such as helium and neon, exhibit exceptional stability due to their complete valence shells. This stability serves as a benchmark for electron configurations.
Principles Guiding Electron Arrangement
Three fundamental principles govern the arrangement of electrons in orbitals:
- Aufbau Principle: Orbitals are filled in order of increasing energy.
- Hund’s Rule: Electrons are distributed across orbitals within an energy level to maximize their spins.
- Pauli Exclusion Principle: No two electrons can have the same set of four quantum numbers.
Sulfur’s Electronic Configuration
Sulfur’s electronic configuration is 1s22s22p63s23p4. This configuration reveals that sulfur has:
- 16 electrons, matching its atomic number.
- 6 valence electrons in the 3s and 3p orbitals.
Implications for Sulfur’s Chemistry
Sulfur’s valence electrons make it highly reactive, forming various types of bonds with other elements. Its ability to share or gain electrons allows it to participate in covalent and ionic interactions.
Sulfur’s six valence electrons give it the potential for diverse bonding scenarios, contributing to its wide range of chemical applications, including fertilizers, pharmaceuticals, and industrial processes.