Title: Efficient Sulfur Production: A Comprehensive Guide To Extraction And Processing Methods
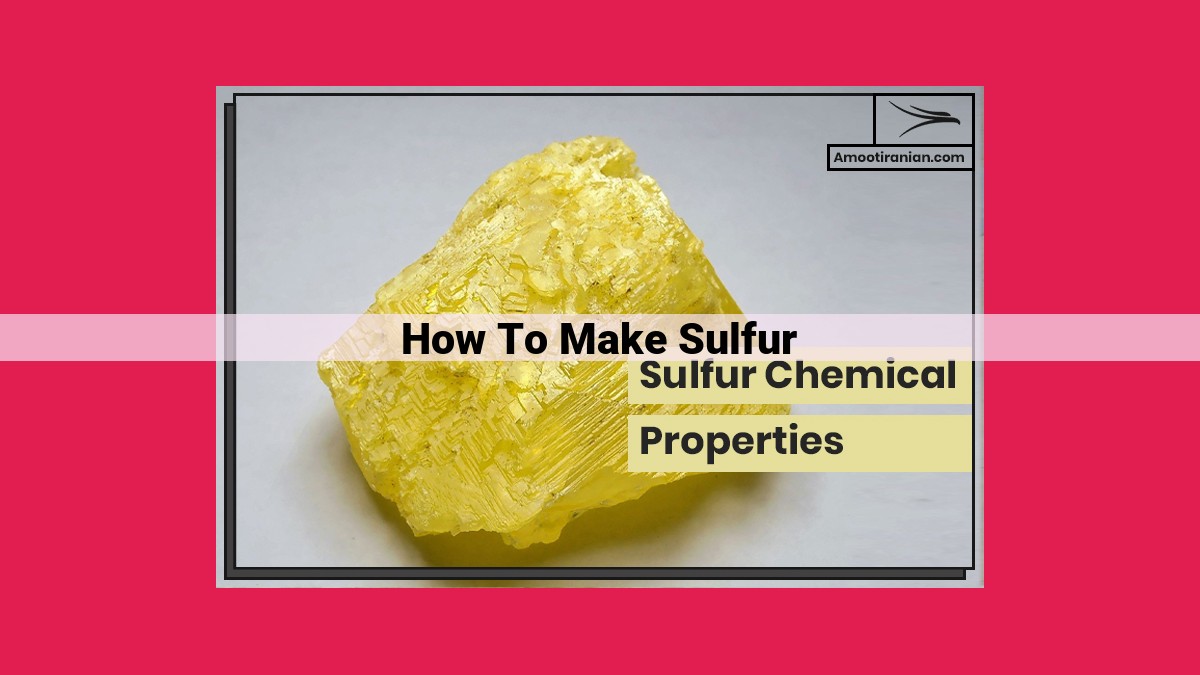
To produce sulfur, various methods are employed depending on its source. Sulfur-containing ores like pyrite can be mined and processed. The Frasch Process involves extracting sulfur from underground deposits using superheated water. The Claus Process chemically converts hydrogen sulfide into sulfur. Hot-Gas Desulfurization and Sulfur Recovery Units remove sulfur from industrial gases and oil refineries. Sublimation and condensation are used to transform solid sulfur into gas and liquid forms, respectively. Finally, precipitation is used to obtain elemental sulfur from solutions.
How to Make Sulfur: A Comprehensive Guide to Sulfur Production
Extraction from Ores: Mining and Processing
For centuries, sulfur has been an indispensable element in various industries, from agriculture to pharmaceuticals. Its production involves a multitude of processes, with ore extraction serving as a cornerstone.
Mining Sulfur Ores
Sulfur-rich ores, such as pyrite and pyrrhotite, are typically found in underground mines or open-pit excavations. Miners excavate these ores using heavy machinery, ensuring efficient extraction and minimal waste.
Processing Ores
Once extracted, the ores undergo a series of processing steps to isolate the elemental sulfur. This includes crushing and grinding the ores into smaller particles, allowing for easier extraction. The crushed material is then roasted, a process where it is heated in the presence of oxygen, causing the sulfur to oxidize and form sulfur dioxide (SO2).
Separation and Collection
The roasted material is subsequently leached with water to dissolve the sulfur compounds. The resulting solution is filtered to remove impurities, after which the sulfur is precipitated out of the solution by adding a reducing agent, such as hydrogen sulfide (H2S). The precipitated sulfur is then collected and further processed to obtain the final product.
Beyond Ore Extraction
While ore extraction is a fundamental step in sulfur production, advancements in technology have led to the development of alternative methods, such as the Frasch process, Claus process, and hot-gas desulfurization. These processes allow for the extraction of sulfur from various sources, including naturally occurring deposits, industrial emissions, and even from oil and gas processing.
The Frasch Process: Extracting Sulfur from Underground
In the heart of the Earth’s depths, where volcanic forces have forged remarkable deposits, lies a valuable element essential for a myriad of industries: sulfur. To harness this subterranean treasure, the Frasch process, a marvel of engineering, has been employed for over a century.
Imagine a battlefield deep within the Earth, where superheated water becomes the weapon of choice. This water, heated to temperatures高达300华氏度, acts as a formidable solvent, ready to dissolve the sulfur trapped within underground deposits.
Through specially designed boreholes, this superheated water is injected with immense pressure into the sulfur-bearing formations. As it penetrates the rock, its heat melts the sulfur, transforming it from a solid into a liquid state. The molten sulfur, eager to escape its subterranean prison, rises up the borehole, carried by the pressurized water.
The key to the Frasch process lies in the precise temperature control. Superheated water dissolves sulfur without damaging the rock formation, ensuring the extraction of pure elemental sulfur. This technique has revolutionized the sulfur industry, making it possible to access vast reserves that were once inaccessible.
As the molten sulfur ascends through the borehole, air is pumped in to separate the sulfur from the water. The sulfur droplets, now freed from their watery confinement, condense into a liquid form. This liquid sulfur is then collected and further processed to meet the demands of various industries.
The Frasch process is a testament to human ingenuity, enabling us to extract an essential element from the depths of our planet. Its efficiency and precision have made it the dominant method for sulfur production, contributing to a wide range of applications, from fertilizers to pharmaceuticals and even gunpowder.
The Claus Process: Recovering Sulfur from Hydrogen Sulfide
In the heart of industrial settings, where sulfur is an indispensable element, the Claus Process stands as a testament to scientific ingenuity. This ingenious method harnesses hydrogen sulfide (H₂S), a pungent gas that is often a byproduct of various industrial processes, and transforms it into elemental sulfur, a crucial resource for myriad applications.
The Claus Process is a multi-step reaction that takes place within dedicated equipment called a Claus Reactor. Like a chemical symphony, the process begins with the introduction of H₂S and a precise amount of oxygen into the reactor. As the mixture heats up, a captivating dance of molecules unfolds, governed by the following chemical equation:
2H₂S + 3O₂ → 2SO₂ + 2H₂O
In this reaction, H₂S undergoes oxidation, relinquishing its hydrogen atoms and forming sulfur dioxide (SO₂) and water (H₂O). The sulfur dioxide, a colorless gas, is then directed to subsequent steps of the process.
However, the journey doesn’t end there. A portion of the sulfur dioxide produced in the first stage is cleverly recycled back into the Claus Reactor, where it engages in a further reaction with H₂S to generate elemental sulfur:
SO₂ + 2H₂S → 3S + 2H₂O
This intricate interplay between oxidation and reduction reactions within the Claus Reactor ensures that nearly 95% of the H₂S is converted into elemental sulfur. The elemental sulfur condenses from the gas stream, solidifies, and is collected, ready to embark on its journey to various industries.
The Claus Process has become an indispensable method for recovering sulfur from industrial gases. Its ability to transform a waste product into a valuable resource not only benefits industries but also contributes to environmental protection by reducing the emission of sulfur-containing gases into the atmosphere.
Hot-Gas Desulfurization: A Sulfur Savior from Industrial Flue Gases
Amidst the industrial landscape, where towering smokestacks release gases into the atmosphere, a quiet revolution is taking place. Hot-gas desulfurization (HGD) technology stands as a guardian of our environment, transforming the sulfur dioxide (SO₂) menace into a valuable resource – sulfur.
In the dance of chemical reactions, HGD harnesses the power of limestone slurry, a calcium-rich mixture. As industrial flue gases, laden with SO₂, pass through this slurry, a magical transformation occurs. The SO₂ reacts with the calcium oxide present in the slurry, forming solid calcium sulfate. This reaction creates a pure stream of sulfur, which is then separated and collected.
The brilliance of HGD lies not only in its environmental benefits but also in its cost-effectiveness. By capturing sulfur from industrial flue gases, it reduces the need for mining new sulfur ores, conserving valuable natural resources. Moreover, the recovered sulfur can be used in a wide range of industrial processes, including the production of sulfuric acid, fertilizer, and paper.
HGD technology has emerged as a beacon of hope in the battle against air pollution. By removing SO₂ from industrial emissions, it improves air quality and protects human health. This innovative process not only safeguards our environment but also provides a sustainable source of sulfur for various industries. As we march towards a greener future, HGD stands tall as a testament to human ingenuity and the relentless pursuit of a cleaner, healthier planet.
Sulfur Recovery Unit: Removing Hydrogen Sulfide from Gas Streams
Gas streams in oil refineries and gas processing plants often contain hydrogen sulfide (H₂S), a toxic and corrosive gas. To prevent environmental and health hazards, it’s crucial to remove H₂S from these streams before further processing. Enter the sulfur recovery unit (SRU), the unsung hero of gas purification.
The SRU: A Sulfur Transformer
An SRU is an intricate system designed to convert H₂S into elemental sulfur. This conversion process not only eliminates H₂S from the gas stream, but also creates a valuable byproduct that can be used in various industries.
How an SRU Works
The SRU employs a series of chemical reactions to transform H₂S into sulfur. The process can be broken down into three main stages:
- Incineration: H₂S-rich gas is burned with controlled amounts of air, converting H₂S into sulfur dioxide (SO₂).
- Reduction: SO₂ reacts with additional H₂S in the presence of a catalyst, reducing it back to elemental sulfur and water vapor.
- Condensation: The hot sulfur vapor is cooled and condensed into liquid sulfur, which is then stored for future use.
The Importance of SRUs
SRUs play a vital role in environmental protection and the sulfur industry. By removing H₂S from gas streams, they prevent the release of harmful emissions into the atmosphere. Moreover, the recovered sulfur is a valuable raw material used in fertilizers, pharmaceuticals, and other products.
The sulfur recovery unit is an essential component of gas processing facilities, ensuring the safe and efficient removal of H₂S from gas streams. Its ability to convert a toxic gas into a valuable byproduct highlights its importance in both environmental protection and the global sulfur industry.
Sublimation: A Unique Transformation of Sulfur
In the realm of sulfur production, sublimation stands as a captivating process that defies the conventional path from solid to liquid. Instead, solid sulfur embarks on an extraordinary journey, bypassing the liquid state and directly assuming a gaseous form.
Imagine a chamber filled with solid sulfur, its crystalline structure reflecting light like a thousand tiny mirrors. As the temperature rises, a peculiar transformation begins. The bonds holding the sulfur atoms together loosen, granting them increased mobility. Instead of melting into a viscous liquid, the sulfur particles defy expectations and break free from their rigid lattice, transforming directly into a cloud of invisible gas.
The process of sublimation is driven by the unique molecular structure of sulfur. The molecules are composed of eight sulfur atoms arranged in a ring-like configuration, known as a crown. This crown-shaped structure allows the sulfur molecules to pack together efficiently, creating a highly volatile substance. As the temperature increases, the vibrational energy within the molecules overcomes the intermolecular forces, causing them to escape their solid confines.
The sublimation of sulfur has practical applications in its purification and recovery. This process is often employed in the Frasch process, where sulfur is extracted from underground deposits using superheated water. The molten sulfur is then sublimed and condensed to remove impurities and yield high-purity sulfur. Sublimation also plays a role in the sulfur recovery units found in oil refineries and gas processing plants, where hydrogen sulfide (H₂S) is converted into elemental sulfur.
Condensation: Transforming Sulfur Gas into Liquid Gold
Once the sulfur gas is released from its subterranean depths, it embarks on a transformative journey through a cooling gauntlet. As the gas ascends, it encounters a symphony of chilled pipes and chambers, coercing it to abandon its ethereal state and condense into a golden liquid.
The cooling process mimics the gentle caress of a summer breeze, gradually coaxing the sulfur gas to relinquish its gaseous freedom. As the temperature plummets, the sulfur molecules lose their kinetic energy, their dance slowing down until they collide and intertwine, forming liquid droplets that lazily cascade down the walls of the cooling vessels.
The condensation phase is a crucial step in the sulfur production symphony, for it liberates the liquid sulfur from its gaseous shackles, allowing it to be further refined and harnessed for a multitude of industrial applications.
Precipitation: A Method for Extracting Elemental Sulfur from Solutions
In the realm of sulfur production, the art of precipitation plays a pivotal role in isolating elemental sulfur from its aqueous confinement. This intricate process involves the careful addition of specific reagents to a sulfur-containing solution, triggering a cascade of chemical reactions that result in the formation of solid sulfur.
The reagents employed in precipitation vary according to the nature of the solution. For solutions rich in hydrogen sulfide (H₂S), the addition of oxidants such as ozone (O₃) or hydrogen peroxide (H₂O₂) initiates a series of oxidation reactions. These reactions transform H₂S into elemental sulfur, which precipitates out of the solution as a finely dispersed solid.
In solutions containing sulfate ions (SO₄²⁻), the precipitation of sulfur can be achieved using reducing agents such as sodium sulfide (Na₂S) or hydrochloric acid (HCl). These reagents convert sulfate ions into H₂S, which subsequently precipitates as elemental sulfur.
The precise conditions, such as temperature, pH, and reagent concentrations, play a critical role in the efficiency of the precipitation process. Optimizing these parameters ensures maximal sulfur recovery and minimizes the formation of undesirable byproducts.
Once the precipitation process is complete, the solid sulfur is separated from the solution through filtration or centrifugation. The resultant sulfur precipitate can be further processed to obtain high-purity elemental sulfur suitable for a wide range of applications, including fertilizer production, sulfuric acid manufacturing, and the vulcanization of rubber.
The precipitation method offers a versatile and efficient means of extracting elemental sulfur from solutions. By carefully controlling the reaction conditions and selecting appropriate reagents, it is possible to optimize sulfur recovery, minimize byproduct formation, and produce high-quality sulfur for industrial applications.