Understanding The Solvent Front In Chromatography: Key To Effective Separation
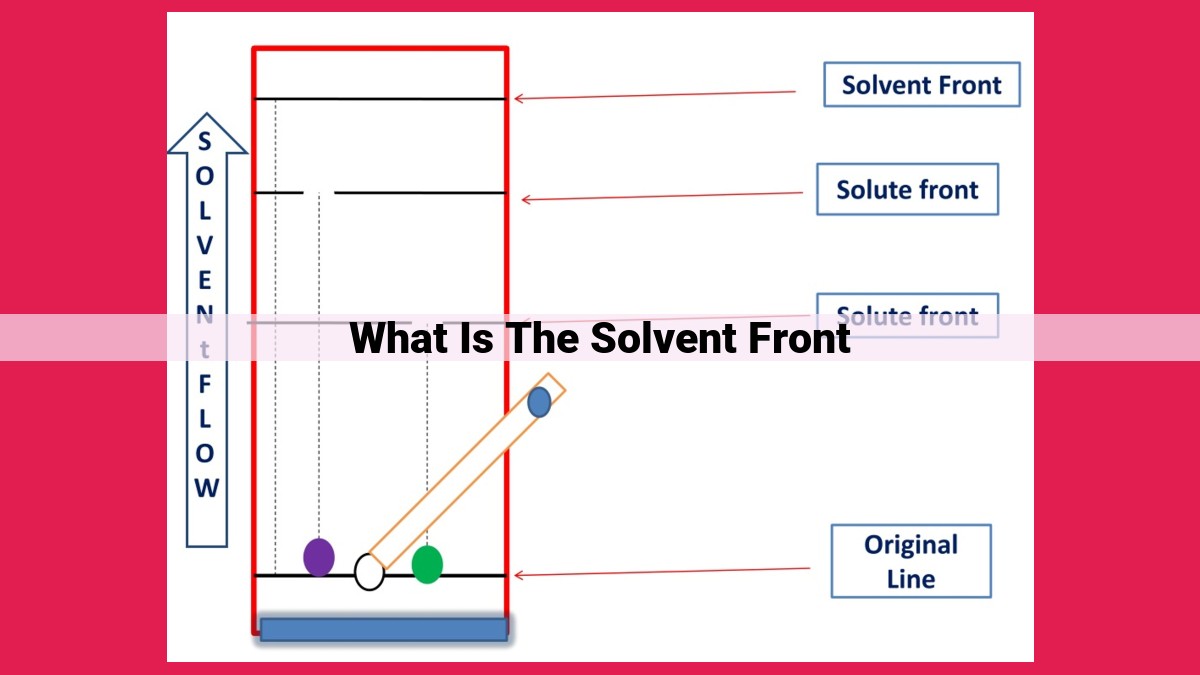
In chromatography, the solvent front is the boundary between the mobile phase and the stationary phase that moves through the column. It represents the point at which the solvent has moved the furthest, carrying with it the sample components. The solvent front plays a crucial role as it determines the separation of different sample components based on their interactions with the mobile and stationary phases.
Understanding the Solvent Front: A Chromatography Odyssey
What is a Solvent Front?
Imagine a chromatography experiment as a race, where the solvent is the runner and the sample components are the obstacles. The solvent front is the leading edge of the solvent as it moves through the chromatography system, clearing a path for the sample components to follow.
The Solvent’s Journey
As the solvent front advances, it encounters sample components with varying affinities for the stationary phase (a solid or liquid that remains in place during the experiment). Components that interact strongly with the stationary phase lag behind, while those with weaker interactions move ahead swiftly.
This differential interaction between the sample components and the stationary phase creates a separation effect, which is the ultimate goal of chromatography. The solvent front acts as a guide, pushing the sample components through the system and enabling their separation based on their unique properties.
Delving into the Stationary Phase: The Immovable Foundation of Chromatography
In the realm of chromatography, the stationary phase plays an indispensable role as the immovable anchor that binds to the dance of molecules within the sample. It is a solid or liquid-coated solid that remains stationary while the liquid or gaseous mobile phase flows past it.
The key function of the stationary phase lies in its ability to selectively interact with different components of the sample. This interaction is governed by various properties of the stationary phase, including its polarity, surface area, and chemical functionality.
A Tapestry of Stationary Phase Materials
Chromatographers have a vast toolbox of stationary phase materials at their disposal, each with distinct properties that tailor them to specific applications. Here are a few commonly encountered types:
- Silica: A polar material with a high surface area that is often used in normal-phase chromatography (where the stationary phase is more polar than the mobile phase).
- Polystyrene-divinylbenzene (PS-DVB): A nonpolar material with a porous structure that is employed in reversed-phase chromatography (where the stationary phase is less polar than the mobile phase).
- Cellulose: A polar material derived from plant fibers that is used in ion-exchange chromatography (where the stationary phase has charged groups that interact with oppositely charged molecules in the sample).
Fine-Tuning Chromatography with Stationary Phase Optimization
The choice of stationary phase is crucial in chromatography, as it directly influences the selectivity and efficiency of the separation. By carefully considering the properties of the sample and the desired separation, chromatographers can select a stationary phase that maximizes interaction with the target molecules while minimizing interference from unwanted components.
In conclusion, the stationary phase is a fundamental component of chromatography, providing the solid foundation upon which the separation of sample components occurs. Its properties and material composition have a profound impact on the selectivity, efficiency, and overall success of the chromatographic analysis.
Exploring the Mobile Phase
- Introduction to the mobile phase and its role in chromatography
- Explanation of the different types of mobile phases used and their impact on separation
Delving into the Mobile Phase: The Vital Fluid in Chromatography
Chromatography is a powerful analytical technique widely used to separate and analyze complex mixtures. At the heart of this process lies the mobile phase, a liquid (or gas) that plays a crucial role in the chromatographic dance.
The mobile phase carries the sample components through the chromatographic system, interacting with the stationary phase and sample components to facilitate their separation. Different mobile phases, with varying properties, can significantly impact the outcome of the chromatographic adventure.
Types of Mobile Phases:
-
Isocratic Mobile Phase: The workhorse of chromatography, an isocratic mobile phase maintains a constant composition throughout the separation. Its stability ensures reproducible results but may limit selectivity.
-
Gradient Mobile Phase: A more versatile explorer, a gradient mobile phase changes its composition gradually over time. This variation allows for finer separation of complex mixtures by adjusting the interactions between sample components and the stationary phase.
Impact on Separation:
The mobile phase’s polarity is a key determinant in its interaction with sample components. Polar mobile phases attract polar sample components more strongly, while nonpolar mobile phases favor nonpolar components. By carefully selecting the mobile phase polarity, chromatographers can tailor the separation to their specific needs.
Another crucial aspect is the mobile phase strength. Stronger mobile phases displace sample components from the stationary phase more effectively, leading to faster elution. Weaker mobile phases, on the other hand, retain sample components longer, providing better separation but requiring longer analysis times.
In summary, the mobile phase is a vital aspect of chromatography, providing the driving force for separation. Its composition and properties can be judiciously optimized to achieve the desired separation and analytical goals. Embarking on this chromatographic exploration, armed with a deep understanding of the mobile phase, empowers scientists to unravel the mysteries of complex mixtures with precision and elegance.
Unveiling the Principles of Chromatography: A Journey Into Separation Science
In the realm of science, chromatography stands as a cornerstone technique, empowering scientists to separate and identify the intricate components of complex mixtures. Chromatography resembles a captivating chase, where molecules embark on a race to outmaneuver each other, ultimately leading to their successful separation.
The fundamental principle of chromatography lies in the differential interactions between molecules with a stationary phase and a mobile phase. Picture this: molecules are swept along by the mobile phase, a liquid or gas that glides through a stationary phase, which is typically a solid or liquid confined within a column or a thin layer.
As the molecules navigate this dynamic environment, their affinity for the stationary phase becomes the deciding factor in their fate. Molecules with a strong attraction to the stationary phase linger behind, slowing their progress, while their less adhesive counterparts swiftly move forward. This selective affinity forms the basis of separation.
The solvent front, a boundary within the mobile phase, marks the front line of solvent movement. It’s like the horizon in a race, progressively advancing as the mobile phase sweeps through the system. Sample components, carried along by this solvent tide, exhibit varying affinities for the stationary phase, causing them to lag behind or surge ahead, ultimately creating distinct bands that reflect their unique characteristics.
The story of chromatography is one of precision and finesse. By carefully selecting the stationary and mobile phases, scientists can orchestrate the separation of molecules with astonishing accuracy. It’s a technique that has revolutionized countless fields, from medicine and forensic science to environmental monitoring and pharmaceutical research.
So, next time you encounter the enigmatic world of chromatography, remember the enchanting dance between molecules, the selective allure of the stationary phase, and the relentless pursuit of separation. It’s a fascinating saga that unfolds with each experiment, revealing the intricate tapestry of our molecular world.
Elucidating Elution and Retention Time: Unraveling the Secrets of Chromatography
In the realm of chromatography, elution and retention time hold pivotal roles in dissecting complex mixtures and identifying their components with precision. Elution, a process akin to unraveling a tapestry, refers to the moment when a sample component emerges from the chromatography system, liberated from its chromatographic journey. This pivotal moment marks the culmination of the separation process, offering a glimpse into the identity of the elusive component.
Retention time, like a meticulous timekeeper, measures the duration that a sample component spends within the chromatography system. It’s a crucial parameter that serves as a unique identifier, akin to a fingerprint, for each component. By meticulously tracking the retention time of each component, scientists can pinpoint their presence and deduce their identity within the complex sample.
Unraveling the Significance of Elution
Elution, the triumphant emergence of a sample component from the chromatography system, holds immense significance in various scientific disciplines. In the field of medicine, it facilitates the detection of minute concentrations of drugs, metabolites, and biomarkers in biological fluids, paving the way for accurate diagnoses and personalized treatments. In environmental science, elution aids in identifying pollutants, contaminants, and other trace compounds, empowering scientists to safeguard our precious ecosystems.
Deciphering the Secrets of Retention Time
Retention time, the unwavering companion of elution, is a valuable tool in the arsenal of analytical chemists. It allows scientists to identify and quantify components within a mixture, even in the face of daunting complexity. Retention time is influenced by an intricate interplay of factors, including the compound’s inherent properties, the nature of the stationary and mobile phases, and the operating conditions employed.
By harnessing the power of retention time, scientists can identify unknown compounds by comparing their retention times to those of known reference standards. This meticulous comparison, akin to a meticulous detective’s work, unravels the identity of the unknown component, providing invaluable insights into its chemical nature.
Concluding Thoughts
Elution and retention time, like two halves of a harmonious whole, are indispensable concepts in the world of chromatography. They provide a roadmap for understanding the intricate separation process, empowering scientists to decipher the secrets of complex mixtures and identify their components with unparalleled accuracy. This knowledge forms the bedrock of countless scientific endeavors, from unraveling the mysteries of the human body to safeguarding our fragile environment.
The Vital Role of Detection in Chromatography
In the intricate world of chromatography, where molecules dance and separate, the detector stands as a sentinel, its keen eye unravelling the secrets hidden within the flowing streams of molecules. It is the final piece in the chromatographic puzzle, transforming the invisible into the discernible, revealing the identities of the enigmatic sample components.
Chromatography is a technique that harnesses the differential interactions between sample components and a stationary and mobile phase to separate and identify them. The detector, a crucial component of the chromatographic system, acts as an electronic sentinel, monitoring the effluent as it emerges from the column. As the separated components elute from the column, the detector measures their physical or chemical properties, translating their presence into quantifiable data.
There exists a myriad of detector types, each with its own unique detection principle and sensitivity. Ultraviolet-visible (UV-Vis) detectors, for instance, harness the absorption of ultraviolet or visible light by the sample components, providing information on their concentration and molecular structure. Fluorescence detectors, on the other hand, detect the emission of light by excited sample molecules, offering high sensitivity and selectivity. Mass spectrometers, the heavyweights of detection, provide intricate details about the molecular structure and elemental composition of the sample components, enabling their unambiguous identification.
The choice of detector depends on the nature of the sample and the analytical goals. For instance, in pharmaceutical analysis, UV-Vis detectors are commonly employed for their versatility and ability to detect a wide range of compounds. In environmental analysis, gas chromatography-mass spectrometry (GC-MS) is often the weapon of choice, providing detailed information on the identity of organic pollutants.
The detector’s role in chromatography is not only to detect the presence of sample components but also to quantify their concentration. The output signal from the detector, whether it be a peak height or area, is directly proportional to the concentration of the analyte in the sample. This quantitative information is crucial for determining the concentration of target analytes in complex mixtures, aiding in decision-making and quality control.
In conclusion, the detector is the unsung hero of chromatography, its keen eye unravelling the secrets of complex mixtures. Through its ability to detect and quantify sample components, the detector provides invaluable insights into the chemical composition of samples, empowering scientists to make informed decisions and gain a deeper understanding of the world around us.