Understanding Secondary Protein Structure: Key Forces And Their Impact
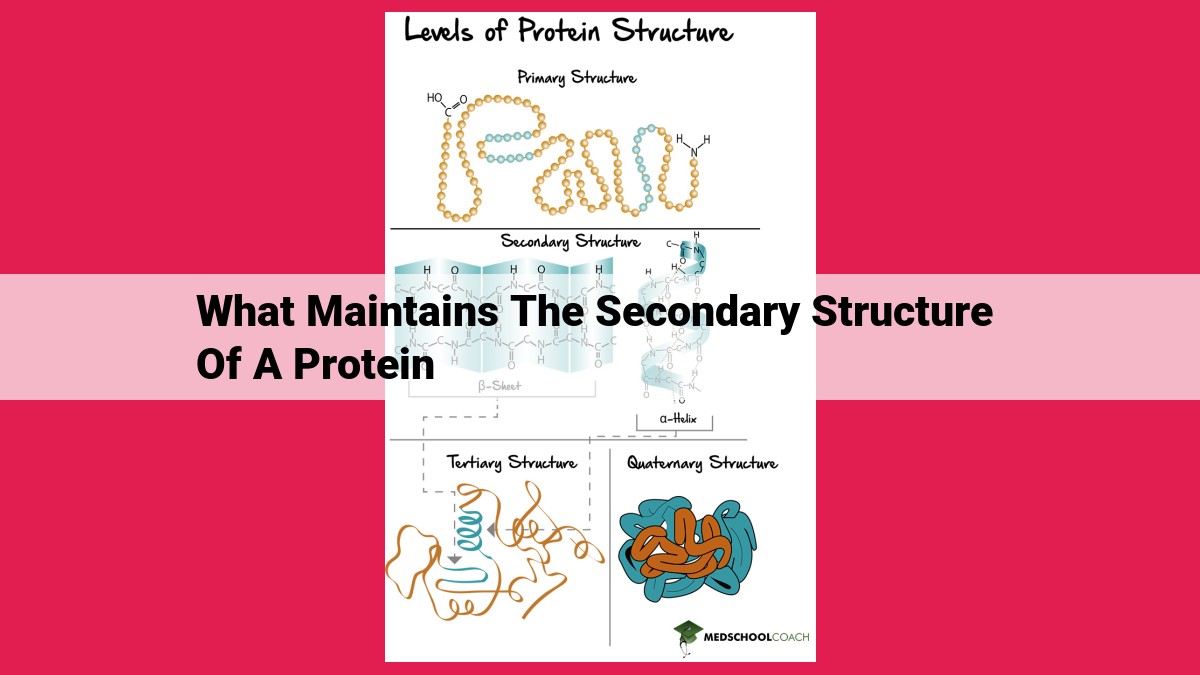
Secondary protein structure is maintained by various forces: hydrogen bonds, van der Waals forces, electrostatic interactions, and disulfide bonds. Hydrogen bonds between backbone atoms stabilize alpha-helices and beta-sheets. Nonpolar side chains interact through van der Waals forces, forming a hydrophobic core. Electrostatic interactions between charged side chains contribute to structural stability. Disulfide bonds between cysteine residues add rigidity and stability. These forces work together to maintain the specific conformations of secondary structure elements, which are crucial for protein function.
The Intricate Architecture of Proteins: Unraveling the Secrets of Secondary Structure
Proteins, the master molecules of life, play a pivotal role in countless cellular processes. Their diverse functions, ranging from enzymatic catalysis to structural support, stem from their intricate architecture. The secondary structure of proteins, a fundamental level of organization, is crucial for understanding how these macromolecules fulfill their biological roles.
At its core, the secondary structure refers to the repeating patterns formed by the backbone of amino acids in a polypeptide chain. These patterns arise due to the formation of hydrogen bonds between the backbone carbonyl oxygen and amide hydrogen atoms. The precise arrangement of hydrogen bonds gives rise to two primary secondary structural elements: the alpha-helix and the beta-sheet.
Alpha-Helices resemble tightly coiled springs, where each amino acid residue forms hydrogen bonds with the fourth residue ahead, creating a spiral staircase. Beta-Sheets, on the other hand, are formed by the parallel or antiparallel alignment of beta-strands, which consist of extended peptide chains held together by hydrogen bonds.
Beyond hydrogen bonds, van der Waals forces also contribute significantly to secondary structure stability. These comparatively weak interactions arise from the attraction between nonpolar side chains of amino acids. The hydrophobic core, formed by the clustering of nonpolar side chains in the interior of the protein, plays a crucial role in maintaining the overall structure by shielding it from the aqueous environment.
Electrostatic interactions between charged side chains, such as positively charged lysine and negatively charged glutamic acid, further contribute to the stability of protein secondary structure. These electrostatic attractions and repulsions balance each other, creating a delicate equilibrium that maintains the desired protein conformation.
Finally, disulfide bonds, covalent bonds formed between the sulfur atoms of two cysteine residues, provide additional stability and rigidity to protein secondary structure. These covalent cross-links restrict conformational flexibility and prevent unfolding, ensuring the protein’s functional integrity.
In summary, the secondary structure of proteins is a complex and dynamic interplay of various forces, including hydrogen bonds, van der Waals forces, electrostatic interactions, and disulfide bonds. These forces collectively maintain the precise arrangement of the polypeptide chain, providing the structural foundation for protein function. Understanding the intricate details of secondary structure is essential for deciphering the mechanisms that govern protein behavior and ultimately, the fundamental processes of life.
Hydrogen Bonds: The Architects of Protein Structure
Introduction:
Proteins, the workhorses of our cells, are intricate molecular machines that perform countless functions essential for life. One key to their remarkable efficiency lies in their secondary structure, which defines the overall shape and stability of protein molecules. Hydrogen bonds, the invisible forces that link atoms together, play a pivotal role in shaping this secondary structure.
Hydrogen Bonds in Action:
Alpha-Helices and beta-sheets are two prevalent secondary structures in proteins. Alpha-helices resemble spiral staircases, with their backbone atoms forming a regular coil. Beta-sheets, on the other hand, resemble pleated sheets, with their backbone atoms arranged in parallel lines.
The secret behind these intricate structures lies in the specific interactions between backbone carbonyl oxygen and amide hydrogen atoms. These atoms form hydrogen bonds, which are attractive forces that stabilize the protein’s structure. In alpha-helices, hydrogen bonds occur between every fourth amino acid, maintaining the helical shape. In beta-sheets, hydrogen bonds form between adjacent strands, connecting them like the rungs of a ladder.
Beyond the Basics:
The strength and orientation of hydrogen bonds depend on the specific amino acids involved. Amino acids with polar side chains (e.g., serine, threonine) can form additional hydrogen bonds with surrounding water molecules, further stabilizing the secondary structure. Nonpolar side chains (e.g., leucine, isoleucine), on the other hand, tend to cluster together to form a hydrophobic core, shielded from water.
Conclusion:
Hydrogen bonds are the unsung heroes of protein structure. They orchestrate the intricate arrangement of amino acids, resulting in the characteristic shapes that define protein function. From the spiraling alpha-helix to the pleated beta-sheet, hydrogen bonds are the invisible architects that shape the molecular machinery of life.
Van der Waals Forces: The Unsung Heroes of Protein Stability
Just like a sturdy bridge relies on intricate forces to stay standing, the stability of proteins depends on a web of interactions, including the influential van der Waals forces.
When proteins fold into their functional shapes, they create a hydrophobic core. This core is a cozy haven for nonpolar side chains, which shun water and prefer to snuggle together. As these nonpolar side chains pack tightly, they create temporary bonds called van der Waals forces.
These fleeting connections may seem insignificant, but together, they form a mighty force field that bolsters the protein’s structure. Imagine a bustling city where countless citizens interact in fleeting but stabilizing ways. Van der Waals forces act as these unseen citizens, holding the protein’s scaffold firm.
By repelling water and forming a hydrophobic haven, nonpolar side chains not only cocoon the protein’s core but also prevent it from dissolving into chaos. These unassuming forces may not be as flashy as covalent bonds, but they play an indispensable role in maintaining the structural integrity of proteins, the workhorses of our cells.
Electrostatic Interactions: The Unsung Heroes of Protein Structure
In the molecular orchestra of proteins, electrostatic interactions play a symphony of forces that shape their intricate structures. These interactions, like magnets of the cellular world, attract positively charged side chains to negatively charged ones, pulling different parts of the protein together like an invisible web.
Charged side chains, like the acidic glutamate and basic lysine, become magnets when they donate or accept protons, gaining or losing a charge. This charge imbalance creates an electrostatic field, attracting oppositely charged side chains and stabilizing the protein’s structure.
For example, consider the alpha-helix, a spiral-shaped protein structure. Here, electrostatic interactions between positively charged side chains at one end of the helix and negatively charged side chains at the other end lock the helix in place like a molecular zipper. These interactions counteract repulsive forces between like-charged side chains, ensuring the helix’s stability.
Disulfide Bonds: The Glue that Holds Proteins Together
In the realm of proteins, the arrangement of amino acids into specific shapes determines their function. These shapes are not merely haphazard folds; rather, they are meticulously maintained by a network of intricate forces, one of which is the mighty disulfide bond.
Formation of Disulfide Bonds
Disulfide bonds are covalent linkages that form between two cysteine residues when the sulfur atoms of their side chains react. This process is catalyzed by an enzyme called protein disulfide isomerase (PDI) and occurs in the oxidizing environment of the endoplasmic reticulum.
Contribution to Structural Stability and Rigidity
Disulfide bonds are renowned for their exceptional strength and stability. They act like molecular bridges, connecting different parts of the protein and preventing it from unraveling. This is particularly critical for proteins that are exposed to harsh conditions, such as enzymes that function in acidic or reducing environments.
Moreover, disulfide bonds play a crucial role in maintaining the rigidity of proteins. By locking different sections of the polypeptide chain together, they prevent excessive flexibility and ensure the proper orientation of functional groups necessary for protein activity.
In summary, disulfide bonds are essential for the structural integrity and stability of proteins. They act as covalent anchors, holding proteins in their functional conformations and enabling them to withstand the rigors of their cellular environments.
Summary of Secondary Structure Maintenance
The intricate dance of forces that orchestrate the secondary structure of proteins is a testament to nature’s engineering prowess. Hydrogen bonds, van der Waals forces, electrostatic interactions, and disulfide bonds intertwine harmoniously, painting a vivid canvas of protein architecture.
-
Hydrogen bonds: The enigmatic hydrogen bond, a chemical handshake between electronegative atoms, plays a pivotal role in stabilizing the alpha-helix and beta-sheet structures. Oxygen atoms and hydrogen atoms form a delicate web, cradling the protein’s backbone in its embrace.
-
van der Waals forces: Nonpolar side chains, like shy dancers at a ball, withdraw from the watery solvent, forming a hydrophobic core. Their gentle nudges contribute to the overall stability of the protein’s structure.
-
Electrostatic interactions: Charged side chains, like magnetic opposites, attract each other across the vast expanse of the protein molecule. These interactions, governed by the laws of electromagnetism, provide the perfect counterbalance to the hydrophobic core.
-
Disulfide bonds: Cysteine residues, wearing their sulfur finery, forge unbreakable disulfide bonds that stitch together distant parts of the protein. These covalent bonds, like sturdy threads, lend rigidity and strength to the protein’s scaffold.
The interplay between these forces is a delicate balancing act, a symphony of molecular interactions that give birth to the remarkable diversity of protein structures. Each force plays a unique role, contributing to the overall stability, solubility, and function of the protein. Understanding these forces is the key to unlocking the secrets of protein’s multifaceted nature.