Unveiling Scientific Principles: Unlocking The Secrets Of Scientific Inquiry And Reliable Knowledge
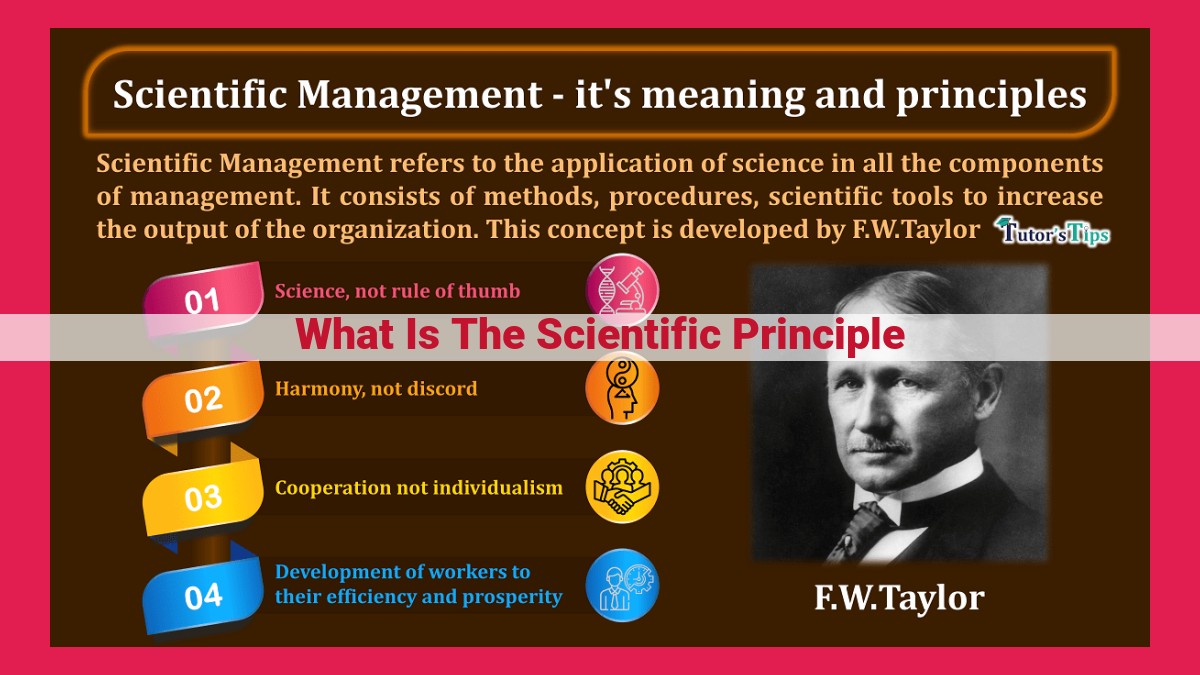
The scientific principle encompasses the fundamental principles and processes that guide scientific investigation. It includes the scientific method, hypothesis formulation, theory building, and experimental design. The scientific principle emphasizes the importance of testability, replication, and peer review to ensure the reliability and validity of scientific knowledge. It involves understanding variables, collecting data, analyzing results, and drawing conclusions based on evidence. By adhering to these principles, scientists strive to make objective observations, form testable explanations, and develop comprehensive theories that explain natural phenomena.
Understanding the Scientific Principle: A Guide to Essential Concepts
Embark on a scientific adventure as we delve into the fundamental principles that govern the world around us. The scientific method, the cornerstone of scientific inquiry, serves as a systematic approach to unraveling the mysteries of nature. It involves observing the world, formulating hypotheses, testing them through experiments, and drawing conclusions based on the gathered evidence.
Through this methodical process, scientists seek to understand the natural world, uncovering its laws and theories. These principles provide explanations for the phenomena we witness, allowing us to predict future events and harness our knowledge for technological advancements.
Hypothesis: Proposing Explanations
In the realm of science, hypotheses stand as the cornerstones of our quest for knowledge. They are proposed explanations for a given phenomenon, serving as testable predictions that drive scientific inquiry.
To fully grasp the essence of a hypothesis, we must first understand its connection to evidence and observations. These building blocks of scientific thought represent the raw data gathered through meticulous investigations. Observations are objective accounts of what is witnessed, while evidence provides support and strengthens these observations.
At the heart of a hypothesis lies its testability. Scientists meticulously craft hypotheses that can be put to the test through experiments. These experiments serve as the crucible of scientific inquiry, providing the critical data that either supports or refutes the hypothesis.
But how do we proceed once a hypothesis is formulated? The next step in the scientific process involves the development of a theory. A theory is a well-supported explanation that encompasses a broad range of observations and experimental findings. It provides a more comprehensive framework for understanding complex natural phenomena.
The relationship between hypotheses and theories is intertwined. A hypothesis serves as a stepping stone towards a more refined theory. As scientists gather more evidence and conduct further experiments, they build upon the initial hypothesis, gradually solidifying it into a robust theory.
It’s important to note that both hypotheses and theories are subject to change and refinement. Science is an iterative process, continuously evolving as new knowledge emerges. The key lies in the willingness to test, modify, and adapt our explanations based on empirical evidence.
Theory: Explaining Natural Phenomena
In the realm of scientific inquiry, theories hold a pivotal position. They serve as well-supported explanations that provide a comprehensive understanding of natural phenomena. Unlike hypotheses, which are tentative explanations, theories have undergone rigorous testing and have accumulated a substantial body of evidence to support their validity.
Scientific theories are built upon a foundation of hypotheses, which are testable propositions that attempt to explain specific observations or phenomena. Through meticulous experimentation and observation, scientists gather data that either supports or refutes these hypotheses. If a hypothesis consistently aligns with empirical evidence, it can gradually evolve into a theory.
The hierarchy of scientific knowledge progresses from hypotheses, to theories, and ultimately to laws. Laws represent the most fundamental principles that describe constant relationships within the natural world. While theories provide explanations for phenomena, laws simply describe how they behave. For instance, the theory of gravity explains why objects fall, while the law of gravity quantifies the force that governs their descent.
The transition from hypothesis to theory is a gradual process that requires a substantial amount of replication, analysis, and peer review. By replicating experiments and collecting data from multiple sources, scientists can verify the reliability of their results and minimize the influence of potential biases. Sophisticated statistical and qualitative methods are then employed to analyze the collected data, allowing scientists to draw meaningful conclusions and refine their theories.
Finally, the process of peer review plays a crucial role in ensuring the credibility of scientific theories. In this rigorous process, experts in the field evaluate research findings, identify potential flaws, and provide feedback. Constructive criticism and external validation are essential for refining theories and establishing their validity within the scientific community.
Law: Describing Natural Relationships
In the realm of science, the laws stand as immutable principles, governing the natural world with an ironclad grip. Scientific laws describe constant relationships between phenomena, providing a solid foundation for our understanding of the universe. These laws are not mere abstract concepts but rather tangible guidelines that shape the fabric of reality.
Unlike theories, which offer explanations for natural phenomena, laws simply describe the observed relationships. They do not delve into the underlying mechanisms but instead capture the essence of these connections. For instance, Newton’s law of universal gravitation states that every particle in the universe attracts every other particle with a force proportional to their masses and inversely proportional to the square of the distance between them. This law predicts gravitational behavior without explaining its cause.
The connection between theories and laws is a testament to the interconnectedness of scientific knowledge. Theories explain why certain relationships exist, while laws describe how they manifest. This synergistic interplay allows scientists to understand and predict the behavior of the natural world.
By defining scientific laws, we gain a deeper appreciation for their role in our scientific understanding. They are not mere descriptions but rather immutable principles that govern the universe. Through their interplay with theories, laws provide a comprehensive framework for comprehending the workings of nature.
Replication: A Cornerstone of Scientific Reliability
In the realm of science, ensuring the accuracy and validity of research findings is paramount. One crucial aspect of this process is replication, the act of repeating an experiment under similar conditions. Replication serves as a cornerstone of scientific reliability, enabling us to verify the consistency of results and minimize the influence of random or systematic errors.
The importance of replication stems from the inherent variability in scientific investigations. Even under seemingly controlled conditions, numerous factors can introduce biases or inconsistencies. These may include variations in equipment calibration, environmental conditions, or the subjective interpretation of data.
By replicating experiments, scientists can identify and address potential confounding variables. If an experiment yields different results when repeated, it suggests that the original findings may have been flawed or influenced by chance factors. Conversely, if the results are consistent across multiple replications, it strengthens the confidence in the validity of the findings.
Another key element in ensuring reliability is the use of controls. Controls are experimental conditions that are identical to the experimental group except for the absence of the independent variable. By comparing the experimental group with the control group, scientists can isolate the effect of the independent variable and rule out alternative explanations for the observed outcomes.
Proper control design and implementation help minimize error and enhance the precision of scientific findings. By replicating experiments with appropriate controls, scientists can increase the likelihood that their results are reproducible and generalizable, ensuring the integrity of the scientific process and the advancement of knowledge.
Variables: The Dynamic Factors in Scientific Investigations
In the realm of scientific exploration, variables play a pivotal role in unraveling the mysteries of the natural world. They are like the dynamic factors that change under different conditions, allowing scientists to observe and analyze how these changes affect the outcome of an experiment.
Defining Variables
A variable is essentially a measurable characteristic that exhibits variation. In other words, it’s a factor that can change from one observation to another. Variables are crucial for scientific inquiry because they enable researchers to identify and control the elements that might influence the results of their experiments.
Types of Variables
There are several types of variables that scientists often encounter:
- Independent variable: This is the variable that the experimenter manipulates to observe its effect on other variables.
- Dependent variable: This is the variable that responds to changes in the independent variable.
- Control variable: This is a variable that is kept constant to eliminate its potential influence on the experiment.
Independent Variables: Driving Change
Independent variables represent the factors that scientists can change to see how they affect the dependent variable. For example, in an experiment investigating the effects of fertilizer on plant growth, the amount of fertilizer applied would be the independent variable.
Dependent Variables: Measuring Outcomes
Dependent variables, on the other hand, represent the measurable outcome of an experiment. They are the factors that scientists expect to change as a result of manipulating the independent variable. In our plant growth experiment, the height of the plants would be the dependent variable.
Control Variables: Isolating Influences
Control variables are essential for ensuring the validity of an experiment. By keeping them constant, scientists can eliminate the possibility that other factors might be affecting the dependent variable. For instance, in the plant growth experiment, the temperature and light conditions would need to be controlled to ensure that they do not influence the results.
Variables are the cornerstone of scientific experimentation. They allow researchers to identify the factors that affect an outcome and to control for those that do not. Understanding the different types of variables and their roles is crucial for conducting rigorous and meaningful scientific investigations. By manipulating and observing variables, scientists can uncover the intricate relationships and patterns that govern the natural world.
Data: The Foundation of Scientific Reasoning
Data is the crucial building block of any scientific inquiry. It serves as the empirical evidence upon which scientists can test their hypotheses and draw meaningful conclusions. The process of collecting valid data is therefore paramount in ensuring the accuracy and reliability of scientific findings.
Qualitative Data vs. Quantitative Data
Depending on the nature of the research question, scientists may collect either qualitative or quantitative data. Qualitative data is non-numerical and typically involves observations, interviews, and textual analysis. It provides rich insights into complex phenomena that may not be easily quantified. In contrast, quantitative data is numerical and involves measurements, surveys, and statistical analysis. It allows for the precise comparison and analysis of variables.
Methods for Data Collection
The choice of data collection method depends on the specific research objectives and the type of data required. Common methods include:
- Observational studies: Scientists observe and record real-world phenomena without manipulating them.
- Experiments: Scientists manipulate variables and observe the resulting changes in a controlled environment.
- Surveys: Scientists collect data from a sample of individuals through questionnaires or interviews.
- Statistical sampling: Scientists randomly select a subset of a population to represent the entire group.
Ensuring Data Validity
To ensure the validity of collected data, scientists adhere to rigorous standards. They minimize bias by using control groups and randomized trials. They also carefully calibrate and validate their instruments to collect accurate measurements.
Data is the lifeblood of scientific inquiry. Through careful collection and analysis, scientists can test hypotheses, draw conclusions, and contribute to our understanding of the natural world. Valid data is the key to unlocking the power of science in advancing human knowledge and improving our lives.
Peer Review: The Gatekeeper of Scientific Validation
In the realm of scientific research, credibility is paramount. Peer review stands as an indispensable sentinel, meticulously scrutinizing and validating the accuracy of scientific findings before they reach the wider public.
The Rigorous Process of Peer Review
Peer review embodies a collaborative process where experts in a particular field independently assess the merits of a scientific study. These reviewers, carefully chosen for their expertise and impartiality, meticulously examine the research methods, data analysis, and conclusions presented in the manuscript. They may request revisions or clarifications to ensure the study meets the highest standards of scientific rigor.
The Significance of Peer Review
The significance of peer review cannot be overstated. It serves as a critical quality control measure, filtering out flawed or biased research from the scientific literature. By subjecting studies to critical review, peer review enhances the trustworthiness and reliability of scientific findings, ensuring that only the most rigorous and well-supported research reaches the public.
Peer review also fosters scientific integrity. By requiring researchers to justify their methods and conclusions to their peers, peer review promotes transparency and accountability within the scientific community. It discourages scientific misconduct and ensures that research findings are based on sound evidence and objective reasoning.
The Impact on Research Quality
Studies have consistently shown that peer-reviewed research is of higher quality than non-peer-reviewed research. Peer review significantly reduces the likelihood of errors, biases, and unsubstantiated claims in scientific publications. It elevates the credibility of scientific findings, making them more likely to be cited and used to inform policy and decision-making.
Peer review is the cornerstone of scientific validation, safeguarding the integrity and reliability of scientific research. It ensures that the public has access to the most accurate and trustworthy scientific information, a fundamental pillar of our knowledge-based society.