Scientific Explanation: The Foundation Of Reliable Knowledge
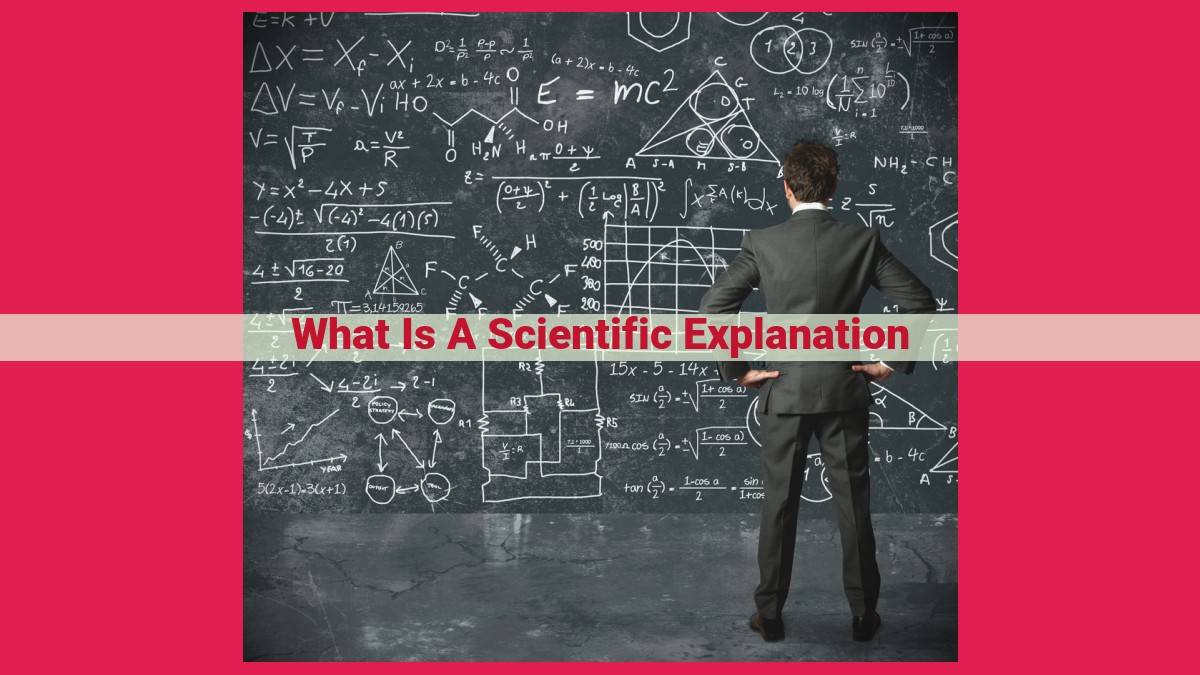
A scientific explanation constructs a logical framework based on observations, data, and evidence. It employs inductive and deductive reasoning to infer generalizations, laws, and theories that unite scientific knowledge. Hypotheses, tested through experiments and research studies, provide empirical validation, while the principles of falsifiability and parsimony guide the evaluation of explanations. This process strengthens the understanding of natural phenomena and ensures the adaptability and reliability of scientific knowledge.
Foundation of Scientific Explanations: Observations
In the realm of science, our quest for understanding the complexities of the world around us begins with observations. Data, evidence, and facts serve as the indispensable building blocks upon which scientific explanations are meticulously constructed.
Data represents the raw information collected through experiments, measurements, or surveys. It provides the foundation for scientific inquiry, allowing researchers to describe the observable patterns and phenomena that they encounter. Evidence, on the other hand, is the interpretation and analysis of data, providing support for or refuting scientific hypotheses. By carefully scrutinizing evidence, scientists strive to uncover the hidden truths that lie beneath the surface of observations.
Facts, the undisputed truths established through rigorous scientific investigation, serve as the cornerstone of scientific knowledge. They represent the collective understanding of the natural world, based on extensive empirical evidence. These facts guide scientists in formulating testable hypotheses and developing comprehensive theories that aim to explain the observed phenomena.
Without observations, science would be merely a collection of abstract ideas devoid of any connection to the tangible world. It is through the patient gathering and meticulous analysis of observational data that scientists lay the groundwork for reliable and fact-based explanations.
Drawing Generalizations: The Power of Inductive Reasoning in Science
In the realm of scientific inquiry, observations are the cornerstone upon which explanations are built. Scientists meticulously gather data, evidence, and facts to paint a clear picture of the natural world. This foundation is crucial for understanding the patterns and relationships that govern our universe.
One of the most fundamental tools scientists employ to make sense of their observations is inductive reasoning. This process involves drawing generalizations and inferences based on patterns and regularities observed in the data. Scientists may notice similarities, connections, or trends among multiple observations, leading them to formulate tentative conclusions.
For instance, after observing numerous instances of objects falling to the ground, scientists generalized that gravity is a universal force that attracts objects toward each other. This generalization forms the basis of a more comprehensive theory of gravitation.
Inductive reasoning allows scientists to move beyond individual observations and identify underlying principles that govern natural phenomena. By synthesizing diverse data points, they can infer relationships and identify patterns that may not be readily apparent from a single observation.
The strength of inductive reasoning lies in its ability to generate new insights and hypotheses that can be further tested and refined. Scientists rely heavily on this approach to advance our understanding of the world and make predictions about future occurrences.
Testing Theories: The Role of Hypotheses
In the intricate tapestry of scientific inquiry, hypotheses serve as the threads that connect observations to theories. They are proposed explanations that can be empirically tested, venturing into the unknown to unravel the mysteries of our world.
Imagine a detective unraveling a complex case. Each clue, each piece of evidence, leads them closer to the truth. In science, hypotheses are similar clues, guiding researchers towards the ultimate revelation—the comprehensive theory that explains the phenomena under investigation.
To formulate a hypothesis, scientists draw upon their observations and inductive reasoning, constructing a tentative explanation that accounts for the patterns they have observed. These hypotheses are not mere guesses; they are testable propositions, crafted to withstand the rigorous scrutiny of experimentation and evidence.
Once a hypothesis has been proposed, it becomes a beacon of discovery, guiding scientists in designing experiments and research studies to test its validity. If the experiments align with the predictions of the hypothesis, its credibility is strengthened. However, if discrepancies arise, the hypothesis is either refined or discarded, giving way to alternative explanations.
The refutability of hypotheses is a cornerstone of scientific inquiry. Unlike dogmatic beliefs, scientific theories must be open to challenge and revision based on new evidence. By subjecting hypotheses to empirical testing, scientists ensure that their theories rest upon a solid foundation of evidence, continually refined and perfected in the pursuit of knowledge.
Establishing Patterns: Laws in Science
In the realm of scientific inquiry, laws reign supreme as principles that distill the essence of consistent patterns observed in nature. They emerge from the crucible of empirical evidence, meticulously gathered through observations, experiments, and meticulous research.
Laws of science transcend the realm of mere descriptions; they possess predictive power. By embodying the underlying relationships and cause-and-effect sequences within natural phenomena, they empower scientists to anticipate future events and behaviors. These laws provide a solid foundation for understanding the intricate workings of our universe.
One captivating example of a scientific law is Newton’s Law of Universal Gravitation. This law eloquently describes the force that attracts any two objects possessing mass. It elegantly encapsulates the universal tendency for objects to gravitate towards one another, with the force’s strength proportional to the product of their masses and inversely proportional to the square of the distance between them.
Another illustrative law is the Law of Conservation of Energy. This law proclaims that the total energy within an isolated system remains constant, providing scientists with a powerful tool to analyze and predict energy transformations in various systems.
Scientific laws are not immutable edicts, chiseled in stone for eternity. Instead, they are subject to revision and evolution as new evidence emerges. This dynamic nature underpins the progress of science, allowing for the refinement and expansion of our understanding of the cosmos.
In conclusion, scientific laws are the cornerstones of our understanding of the natural world. They distill consistent patterns observed in nature, enabling us to predict future events and behaviors. Their empirical foundation and predictive power make them indispensable tools for unraveling the mysteries of our universe.
Unifying Concepts: Scientific Theories
In the realm of scientific inquiry, theories stand as towering pillars of knowledge, unifying diverse observations and laws under their comprehensive umbrella. Theories provide the grand narratives that encompass all that science has discovered about a particular aspect of the natural world.
A scientific theory is more than just a collection of facts or laws; it is a cohesive framework that explains why and how these phenomena occur. It synthesizes disparate pieces of the puzzle, revealing the underlying unity and coherence of nature.
Theories have the power to predict future events and guide new discoveries. They serve as roadmaps for scientific exploration, helping scientists navigate the uncharted territories of the unknown. By providing comprehensive explanations, theories empower us to understand and predict the workings of our vast and intricate world.
Empirical Validation: The Bedrock of Scientific Truths
In the realm of science, evidence reigns supreme as the arbiter of knowledge. Observations, painstakingly collected from the natural world, provide the foundation upon which scientific explanations are built. Through inductive reasoning, scientists draw generalizations, mapping out the patterns that shape our universe. But it is in the crucible of empirical validation that these generalizations are tested and refined.
Scientific progress hinges on the rigorous testing of theories and hypotheses. Hypotheses, proposed as plausible explanations, serve as stepping stones towards unraveling the complexities of nature. Scientists design experiments, conduct research studies, and make observations to meticulously gather evidence for or against their hypotheses. It is this empirical scrutiny that separates science from mere speculation.
The results of these empirical investigations form the cornerstone of scientific knowledge. Observations, experiments, and research studies provide the vital data that either support or refute theories. Theories that withstand repeated empirical challenges gain credibility, while those that fail to account for the evidence are discarded. Through this rigorous process, science self-corrects, ensuring that explanations accurately reflect the workings of the natural world.
The iterative nature of evidence-based inquiry ensures that scientific explanations are continually refined and improved. Scientists constantly seek out new evidence to challenge existing theories and expand our understanding. This relentless pursuit of knowledge drives scientific progress forward, pushing the boundaries of human comprehension deeper and deeper into the mysteries of the universe.
In conclusion, empirical validation stands as the unwavering foundation of scientific knowledge. Through meticulous observations, rigorous testing, and unyielding pursuit of evidence, science ensures that its explanations are anchored in the reality of the natural world. It is this relentless quest for empirical truth that has propelled humanity to the forefront of understanding and empowered us to shape our destiny in harmony with the laws that govern our universe.
Testability and Refutability: The Bedrock of Scientific Progress
The Essence of Falsifiability
In the realm of science, refutability stands as a fundamental tenet. It asserts that a hypothesis or theory should be open to scrutiny and capable of being disproven through empirical evidence. Unlike dogma or belief, which seek to shield themselves from challenge, scientific claims must withstand the rigors of testing.
The Importance of Testable Hypotheses
For a hypothesis to have scientific merit, it must be testable. This means that it can be formulated into a clear and falsifiable prediction. Scientists design experiments or conduct research studies to collect data that can either support or refute the hypothesis. The goal is not to prove the hypothesis true but rather to test its validity and determine whether it can withstand empirical scrutiny.
Refutability: The Engine of Progress
Science thrives on the principle of refutability. By subjecting hypotheses and theories to rigorous testing, scientists can weed out those that fail to align with observable data. This process of elimination drives scientific progress forward. If a hypothesis is repeatedly tested and consistently falsified, it is eventually abandoned. In contrast, theories that survive multiple tests gain support and become more widely accepted within the scientific community.
The Role of Observations and Experiments
Observations provide the foundation for scientific inquiry. Scientists make careful and systematic observations of the world around them, collecting data that can be used to formulate hypotheses and theories. Experiments serve as controlled tests that allow scientists to isolate variables and verify or refute hypotheses under specific conditions.
The Principle of Falsifiability: A Legacy of Karl Popper
The principle of falsifiability was championed by the philosopher Karl Popper in the mid-20th century. Popper argued that scientific knowledge is not absolute but rather tentative and subject to revision as new evidence emerges. He believed that true scientific progress lies in the constant testing and refining of ideas, a process that can only occur if hypotheses and theories are capable of being falsified.
In summary, refutability is an indispensable aspect of the scientific method. It ensures that hypotheses and theories are open to rigorous testing, that they can be disproven by empirical evidence, and that scientific progress is driven by the relentless pursuit of knowledge and the rejection of dogma. By embracing the principle of falsifiability, science remains a powerful tool for understanding the complexities of our world.
Simplicity and Clarity: The Principle of Parsimony
In the realm of scientific inquiry, where the quest for knowledge drives us to unravel the mysteries of the natural world, the principle of parsimony plays a pivotal role in shaping our explanations. This principle, often referred to as Occam’s Razor, advocates for the simplest and most economical theories that can adequately account for all observations.
The principle of parsimony is rooted in the belief that simplicity is often a virtue in science. When faced with multiple explanations for a given phenomenon, scientists naturally gravitate towards the one that requires the fewest assumptions and postulates. This preference for simplicity stems from the recognition that complex theories are more susceptible to errors and inconsistencies.
Consider the classic example of celestial motion. In the 16th century, the predominant theory, known as the Ptolemaic system, proposed a complex arrangement of epicycles and deferents to explain the observed movements of the planets. In contrast, Copernicus’s heliocentric model, which placed the sun at the center of the solar system, offered a far simpler explanation. By adopting the heliocentric model, scientists were able to eliminate a multitude of unnecessary assumptions and complexities, leading to a more elegant and accurate understanding of planetary motion.
The principle of parsimony also emphasizes clarity in scientific explanations. A good theory should not only be simple but also clearly articulate the underlying mechanisms and relationships. Theories that are overly convoluted or ambiguous make it difficult for scientists to communicate their findings and for the public to understand the implications.
In practice, the principle of parsimony guides scientists in selecting among competing hypotheses. When presented with multiple hypotheses that make similar predictions, scientists will often favor the one that is more parsimonious. This approach helps to prevent overfitting, which occurs when a theory is too complex and explains more than what is necessary, potentially leading to inaccurate conclusions.
It is important to note that the principle of parsimony is not a strict rule but rather a guiding principle. Scientists do not always choose the simplest explanation simply because it is simple. Ultimately, the best theory is the one that is supported by the most compelling evidence and provides the most accurate predictions.
However, the principle of parsimony has proven to be an invaluable tool in scientific research. By advocating for simplicity and clarity, it promotes rigor, objectivity, and communication in the pursuit of scientific knowledge.