Understand Residence Time: Key To System Optimization And Process Efficiency
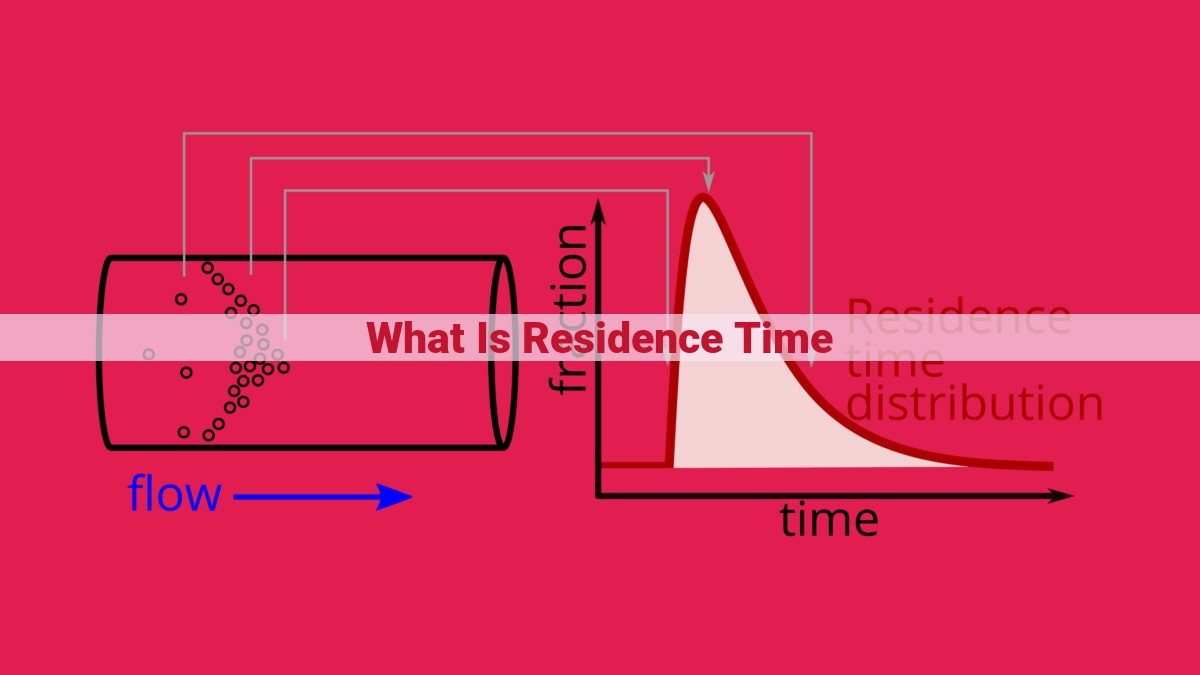
Residence time is the average time a molecule or particle spends within a system. It is a crucial parameter in understanding system behavior, especially in chemical reactors, environmental processes, and wastewater treatment. Different types of residence time exist, depending on the system’s configuration (open or closed), and its operating conditions (steady-state or non-steady-state). Calculating residence time involves considering the system’s volume and flow rate. Related concepts include mean residence time, variance, and dispersion, which provide insights into the distribution of residence times. Residence time is widely used to optimize system performance, control reactions, and design efficient processes.
What is Residence Time?
In a world where time is of the essence, understanding residence time becomes paramount. It’s the duration a substance or entity spends within a system, be it a reactor, a lake, or even your favorite coffee mug. This concept is crucial for comprehending system behavior, as it influences everything from chemical reactions to environmental processes.
Residence time provides a window into how systems evolve over time. It’s like the stopwatch of the substance’s journey, revealing its interactions with the system and the dynamics that shape its fate. Understanding this concept empowers us to optimize systems and harness their potential.
Types of Residence Time
Residence time is a key concept in understanding system behavior. It refers to the average time that a component or entity spends within a particular system or process. Depending on the nature of the system, residence time can vary significantly. In this section, we’ll explore the different types of residence time and their implications:
Open System Residence Time
An open system is one that exchanges mass or energy with its surroundings. In this type of system, the residence time is typically not constant. Instead, it can fluctuate depending on the inflow and outflow rates. For example, in a chemical reactor, the residence time of reactants may vary based on the rate at which reactants are added to the reactor and the rate at which products are removed.
Closed System Residence Time
A closed system is one that does not exchange mass or energy with its surroundings. In this type of system, the residence time is constant because the total amount of material within the system remains constant. For instance, in a batch reactor, the residence time is equal to the total reaction time because no material enters or leaves the reactor during the reaction.
Steady-State Residence Time
Steady-state residence time is a special case of residence time that occurs when the inflow and outflow rates of a system are constant. In this state, the residence time of components within the system remains constant over time. Steady-state residence time is often used to characterize systems that operate continuously, such as chemical reactors and wastewater treatment plants.
Non-Steady-State Residence Time
Non-steady-state residence time occurs when the inflow and outflow rates of a system are not constant. In this case, the residence time of components within the system varies over time. Non-steady-state residence time is often observed during the start-up or shut-down of a system, or when there are sudden changes in the inflow or outflow rates.
Calculating Residence Time: Unlocking the Secrets of System Behavior
Understanding residence time is crucial for deciphering the dynamics of a system. It’s a concept that measures the average time spent by a substance or component within a system.
Calculating residence time empowers us to optimize various processes and systems. In an open system, where substances enter and exit continuously, residence time is calculated using the formula:
Residence time = Volume of the system / Volumetric flow rate
For instance, in a chemical reactor, residence time determines the extent of reaction and product yield.
In a closed system, where no exchange of mass occurs with the surroundings, residence time is calculated differently. The formula becomes:
Residence time = Amount of substance in the system / Mass flow rate
This calculation is vital in systems like wastewater treatment plants, where residence time influences the removal efficiency of pollutants.
In steady-state, where the system’s properties remain constant over time, residence time provides valuable insights into system behavior. The formula for steady-state residence time is:
Residence time = Volume of the system / Flow rate
This calculation helps engineers optimize residence time to achieve desired process outcomes, such as maximizing reaction rates or minimizing wastewater contamination.
Related Concepts: Delving into Residence Time Parameters
In addition to residence time, several related concepts provide deeper insights into the dynamics of a system:
Mean Residence Time:
The average time spent by a particle or molecule dentro system. It represents the expected duration for which an entity occupies the given space.
Variance of Residence Time:
A measure of the deviation from the mean residence time. It indicates the spread or variability in the time particles spend within the system. A higher variance implies a greater spread.
Dispersion:
A quantitative expression of the distribution of residence times within a system. It describes how widely spread the particle trajectories are. High dispersion indicates a large spread, while low dispersion indicates a narrow distribution.
These parameters provide a comprehensive picture of the residence time characteristics of a system, helping us understand the movement of particles, optimize reactor performance, and gain insights into system dynamics.
Applications of Residence Time: A Journey Through Diverse Fields
Residence time, a crucial metric in understanding system behavior, finds myriad applications across diverse fields, ranging from the intricate realm of chemical engineering to the vital concerns of environmental science and the efficient management of wastewater treatment plants.
In the world of chemical engineering, residence time plays a pivotal role in the design and optimization of reactors. By carefully manipulating this parameter, engineers can control the extent of chemical reactions, ensuring optimal conversion rates and product yields. In environmental science, residence time is essential for assessing the fate and transport of pollutants in aquatic ecosystems. Researchers utilize this concept to understand how pollutants behave in rivers, lakes, and estuaries, helping to devise effective remediation strategies.
Wastewater treatment plants rely heavily on residence time to optimize the removal of pollutants from wastewater. By carefully designing treatment systems to provide the appropriate residence time, engineers can ensure that microorganisms have sufficient time to break down organic matter and pathogens, delivering clean and safe water back into the environment.
Furthermore, residence time is a valuable tool in the field of hydrology, where it is used to estimate the time it takes for water to travel through a specific section of a river or aquifer. This information is crucial for managing water resources, understanding flood risks, and designing effective water delivery systems.
In the domain of bioreactors, residence time is a critical parameter for optimizing microbial growth and product formation. By controlling the residence time, researchers can manipulate the population dynamics and metabolic activities of microorganisms, leading to enhanced productivity and efficiency.
In summary, residence time is a versatile and invaluable concept that finds applications in a vast array of fields, spanning chemical engineering, environmental science, wastewater treatment, hydrology, and bioreactors. Understanding and optimizing residence time is essential for maximizing system performance, ensuring environmental protection, and unlocking new frontiers in scientific research and technological innovation.
Factors Affecting Residence Time
Flow Rate:
The flow rate of the fluid or gas through the system plays a critical role in determining residence time. Higher flow rates result in shorter residence times as the fluid moves more swiftly through the system. Conversely, lower flow rates lead to longer residence times as the fluid spends more time within the system.
Volume:
The volume of the vessel or reactor also influences residence time. A larger volume provides more space for the fluid to accumulate, resulting in longer residence times. On the other hand, a smaller volume leads to shorter residence times as the fluid has less space to disperse.
Reactor Design:
The design of the reactor or vessel can significantly affect residence time. Factors such as baffling, agitation, and internal configurations can influence the fluid flow patterns and mixing characteristics. Optimized reactor design aims to promote uniform flow and minimize dead zones, thus maximizing residence time and system efficiency.
Optimizing Residence Time for Enhanced System Performance
In the realm of systems analysis, residence time plays a pivotal role in understanding and controlling system behavior. Residence time refers to the average amount of time that a component or particle spends within a system. By optimizing residence time, we can harness its potential to enhance system performance and efficiency.
Imagine a chemical reactor that transforms reactants into products. The residence time of reactants in the reactor determines the extent of their reaction. Optimizing residence time ensures that reactants spend sufficient time within the reactor for complete conversion, maximizing product yield and minimizing unreacted reactants.
In another scenario, consider a wastewater treatment plant. Residence time in the treatment tanks allows contaminants to degrade or settle. Optimizing residence time ensures that contaminants remain in the tanks long enough for effective treatment, meeting environmental discharge standards while minimizing the treatment process time.
Various factors influence residence time, such as flow rate, volume, and reactor design. By manipulating these factors, we can optimize residence time. For instance, increasing the flow rate in an open system reduces residence time, while decreasing the reactor volume in a closed system increases residence time.
Optimizing residence time also involves considering mean residence time, variance of residence time, and dispersion. These concepts provide insights into the distribution of residence times within the system. By minimizing variance and dispersion, we can achieve a uniform residence time for all components, ensuring consistent system performance.
In summary, optimizing residence time is a powerful tool for enhancing system performance and efficiency. By understanding the factors that affect residence time and applying it to various fields, we can tailor systems to meet specific requirements, maximizing outputs, minimizing waste, and ensuring optimal system operation.