Regiochemistry: Predicting Reaction Sites In Organic Chemistry
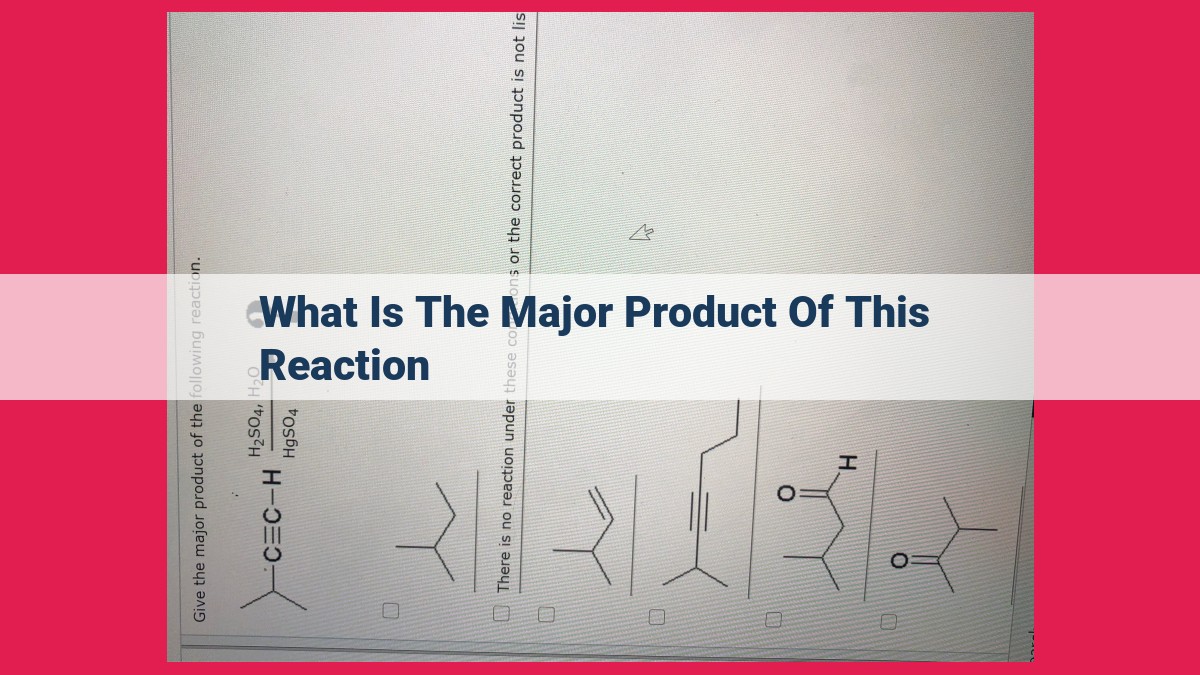
In organic chemistry, regiochemistry refers to the prediction of which position in a molecule a reaction will occur. Markovnikov’s rule predicts that in alkene addition reactions, the carbocation (positive ion) forms on the carbon with the most hydrogens, leading to the major product. However, Anti-Markovnikov’s rule applies in certain conditions, such as reactions with electron-withdrawing groups or specific reagents, resulting in a different regiochemical outcome. Understanding regiochemistry enables chemists to predict major products and design synthetic pathways to achieve desired outcomes.
- Definition and significance of regiochemistry in predicting reaction outcomes
The Enigma of Regiochemistry: Unraveling the Secrets of Chemical Reactions
In the realm of chemistry, one of the most captivating concepts is regiochemistry. It’s a puzzle that guides us in predicting the outcome of chemical reactions, revealing the intricate dance of atoms and molecules. Imagine yourself as a chemist, standing at the threshold of an unknown reaction. With the principles of regiochemistry as your compass, you navigate the chemical landscape, predicting which atoms will embrace and form new bonds, while others remain aloof.
Regiochemistry revolves around the concept of selectivity. It’s like choosing your favorite child; the reaction chooses the most stable product, the one that nature prefers. This preference arises from the inherent stability of certain intermediate structures, called carbocations. Carbocations are positively charged carbon atoms that serve as temporary waypoints in chemical reactions. Just like a wobbly bridge, some carbocations are more stable than others, and this stability plays a crucial role in determining the final product of the reaction.
Markovnikov’s Rule: A Guiding Light in Predicting Reactions
In the realm of organic chemistry, regiochemistry plays a pivotal role in determining the outcome of reactions. It’s all about predicting which atoms or groups will react with each other and where.
One of the most fundamental concepts in regiochemistry is Markovnikov’s Rule. This rule, named after the Russian chemist Vladimir Markovnikov, provides a general guideline for alkene addition reactions (reactions where an atom or group is added to a double bond between carbon atoms).
Markovnikov’s Rule states that:
*In the addition of an unsymmetrical reagent to an unsymmetrical alkene, the _new_ bond will form between the _carbon with the most hydrogens_ and the _atom or group that adds_.
In simpler terms, the more substituted carbon atom in the alkene reacts with the electrophilic part of the reagent, while the less substituted carbon atom reacts with the nucleophilic part.
The Stability of Carbocations: A Behind-the-Scenes Player
To understand why Markovnikov’s Rule works, we need to delve into the world of carbocations. A carbocation is a positively charged carbon atom. They’re like the unsung heroes of organic chemistry, playing a crucial role in determining the regiochemistry of reactions.
According to Markovnikov, the more substituted carbon of an alkene is more likely to form a carbocation. This is because the alkyl groups (CH3, CH2CH3, etc.) attached to the carbocation can donate electrons through a process called hyperconjugation. This stabilizes the carbocation and makes it more likely to form.
In contrast, a less substituted carbocation is less stable. It has fewer alkyl groups to donate electrons, which makes it more reactive and more likely to react with the nucleophilic part of the reagent.
Putting It All Together: Predicting Regioselectivity
So, how do we use Markovnikov’s Rule and carbocation stability to predict the outcome of alkene addition reactions?
- Identify the unsymmetrical alkene and reagent: First, pinpoint the alkene and the reagent that’s adding to it.
- Determine the more substituted carbon: Use your knowledge of carbocation stability to identify the carbon with the most alkyl groups attached.
- Predict the new bond formation: The new bond will form between the more substituted carbon of the alkene and the electrophilic part of the reagent. The less substituted carbon will react with the nucleophilic part.
By following these steps, you can predict the regioselectivity (the preference for one reaction pathway over another) of alkene addition reactions with remarkable accuracy. This knowledge is essential for designing and optimizing organic synthesis routes.
Anti-Markovnikov’s Rule: An Exception to the Norm
When predicting the regiochemistry of alkene addition reactions, Markovnikov’s rule is often our go-to guide. But there’s a sneaky exception to this rule, known as Anti-Markovnikov’s rule.
Anti-Markovnikov’s rule applies under specific conditions. One key factor is the presence of electron-withdrawing groups (EWGs) attached to the alkene. These EWGs, like halogens, carbonyl groups, or nitrile groups, pull electrons away from the double bond.
Another factor that favors Anti-Markovnikov’s rule is the use of certain reagents. Peroxyacids (RCO3H) and oxymercuration-demercuration (Hg(OAc)2-NaBH4) are reagents that tend to react via an anti-Markovnikov pathway.
Why does Anti-Markovnikov’s occur? It’s all about carbocation stability. When EWGs are present, they stabilize the carbocation that forms during the reaction. This makes the Anti-Markovnikov product, with the carbocation on the less substituted carbon, more favorable.
Similarly, peroxyacids and oxymercuration-demercuration reagents create carbocations that are more stable when they form on the less substituted carbon. This steers the reaction towards the Anti-Markovnikov product.
So, when should you consider Anti-Markovnikov’s rule? Keep an eye out for electron-withdrawing groups and specific reagents like peroxyacids or oxymercuration-demercuration. By understanding these exceptions, you’ll be a regiochemistry wizard, able to predict the outcome of these reactions with confidence.
Carbocation Stability and Regioselectivity
When talking about regiochemistry, the stability of carbocations plays a pivotal role in determining the outcome of reactions. Carbocations are positively charged carbon ions formed as intermediates in many organic reactions. Their stability depends on the number of alkyl groups attached to the positively charged carbon.
Tertiary vs. Secondary vs. Primary Carbocations
Carbocations can be classified as primary, secondary, or tertiary. Tertiary carbocations have three alkyl groups attached to the positively charged carbon, secondary carbocations have two, and primary carbocations have only one. The greater the number of alkyl groups, the more stable the carbocation. This is because alkyl groups donate electrons to the positively charged carbon, reducing its charge and increasing its stability.
Stability and Regioselectivity
The stability of carbocations directly influences the regiochemical outcome of reactions. In general, the more stable carbocation formed, the more likely it is to be the major product. This happens because the reaction will proceed through the pathway that leads to the most stable carbocation.
For example, in the addition of hydrogen bromide to an alkene, Markovnikov’s rule predicts that the hydrogen will add to the carbon with the most alkyl groups, forming the more stable carbocation. This is because the carbocation with more alkyl groups is more stable, making the reaction more favorable.
By understanding carbocation stability, chemists can predict the regiochemical outcome of reactions and design synthetic pathways accordingly. This knowledge is essential for controlling the regioselectivity of reactions and obtaining the desired products.
Predicting the Regiochemical Outcome of Reactions
In chemistry, understanding regiochemistry is crucial for predicting the outcome of reactions. It’s like having a crystal ball that gives us a glimpse into the future of our chemical experiments. By using established rules and principles, we can anticipate which products will form and in what proportions. Let’s dive into the secrets of regiochemistry and see how it helps us navigate the unpredictable world of reactions.
Markovnikov’s Rule: A Guiding Light
One of the most important tools in our regiochemical tool kit is Markovnikov’s rule, which states that in the addition of an unsymmetrical reagent to an alkene, the positive portion of the reagent (usually a proton or an electrophile) will add to the carbon atom that has the most hydrogen atoms attached. This rule stems from the formation of a more stable carbocation, which is a key intermediate in many reactions.
Anti-Markovnikov’s Rule: An Exception to the Rule
However, not all reactions follow Markovnikov’s rule. In certain cases, the Anti-Markovnikov’s rule comes into play. This rule states that the positive portion of the reagent adds to the carbon atom with the fewest hydrogen atoms. This exception often occurs when there are electron-withdrawing groups present on the alkene or when specific reagents are used. Understanding the conditions that favor Anti-Markovnikov’s addition allows us to predict the outcome of these reactions accurately.
Carbocation Stability: The Key to Regioselectivity
Carbocation stability is at the heart of regiochemical predictions. More stable carbocations are formed as intermediates in reactions, which in turn determines the regioselectivity of the reaction. For example, tertiary carbocations are more stable than secondary carbocations, which are more stable than primary carbocations. By understanding the relative stabilities of different carbocations, we can predict the regiochemical outcome of reactions involving carbocation intermediates.
Putting It All Together: Predicting the Major Product
Armed with Markovnikov’s and Anti-Markovnikov’s rules, as well as an understanding of carbocation stability, we can now predict the major product of a reaction. By considering these factors, we can determine the regioselectivity of the reaction and identify which product is most likely to form. This knowledge is essential for designing synthetic pathways and optimizing reaction conditions to achieve the desired outcome.
Practical Applications in Synthesis
Understanding regiochemistry is not just an academic exercise; it has practical applications in organic synthesis. By controlling the regioselectivity of reactions, chemists can create specific molecules with the desired properties. For example, in the synthesis of pharmaceuticals, regiochemistry plays a crucial role in determining the biological activity of the final product. By understanding and applying regiochemical principles, chemists can develop more efficient and cost-effective synthetic methods.
Unlocking the secrets of regiochemistry is like having a superpower in the world of chemistry. By using established rules and understanding the underlying principles, we can predict the outcome of reactions and design synthetic pathways with precision. So, next time you’re faced with a chemical conundrum, remember the power of regiochemistry and let it guide you towards the desired outcome.
Practical Applications in Synthesis
- How understanding regiochemistry aids in designing synthetic pathways
- Examples of reactions where regioselectivity is crucial for desired outcomes
Practical Applications of Regiochemistry in Synthesis
Understanding regiochemistry plays a pivotal role in the design of synthetic pathways, enabling chemists to control the regioselectivity of reactions and achieve desired outcomes. One of the most prominent examples of this is the hydroboration-oxidation reaction, a versatile method for introducing hydroxyl groups into alkenes. In this reaction, the regioselectivity can be tailored by carefully choosing the hydroborating agent. For instance, using a sterically hindered hydroborating agent favors the formation of the more substituted alcohol, in accordance with Markovnikov’s rule.
Another application of regiochemistry in synthesis is in carbocation rearrangements. Carbocations are highly reactive intermediates that can undergo rearrangements to form more stable carbocations. By understanding the stability of different carbocations, chemists can design reactions that proceed through the desired rearrangement pathway, leading to the formation of specific products. For example, the pinacol rearrangement relies on the rearrangement of a 1,2-diol to form a ketone or aldehyde. By controlling the regiochemistry of the initial diol formation, the desired product can be selectively obtained.
Regiochemistry also plays a crucial role in cycloaddition reactions, which involve the formation of cyclic compounds from two or more unsaturated molecules. A classic example is the Diels-Alder reaction, where the regiochemistry of the cycloaddition is influenced by the electronic properties of the diene and dienophile. By understanding these electronic effects, chemists can design cycloaddition reactions to selectively form the desired regioisomer.
In summary, regiochemistry serves as a guiding principle for chemists in the design of synthetic pathways. By controlling the regioselectivity of reactions, chemists can selectively obtain the desired products, optimize reaction efficiency, and avoid undesired side reactions. The practical applications of regiochemistry extend across various organic synthesis methodologies, enabling chemists to harness the power of regioselectivity to create complex and valuable molecules.