Quantifying Heat: A Comprehensive Guide To Heat Measurement Techniques And Applications
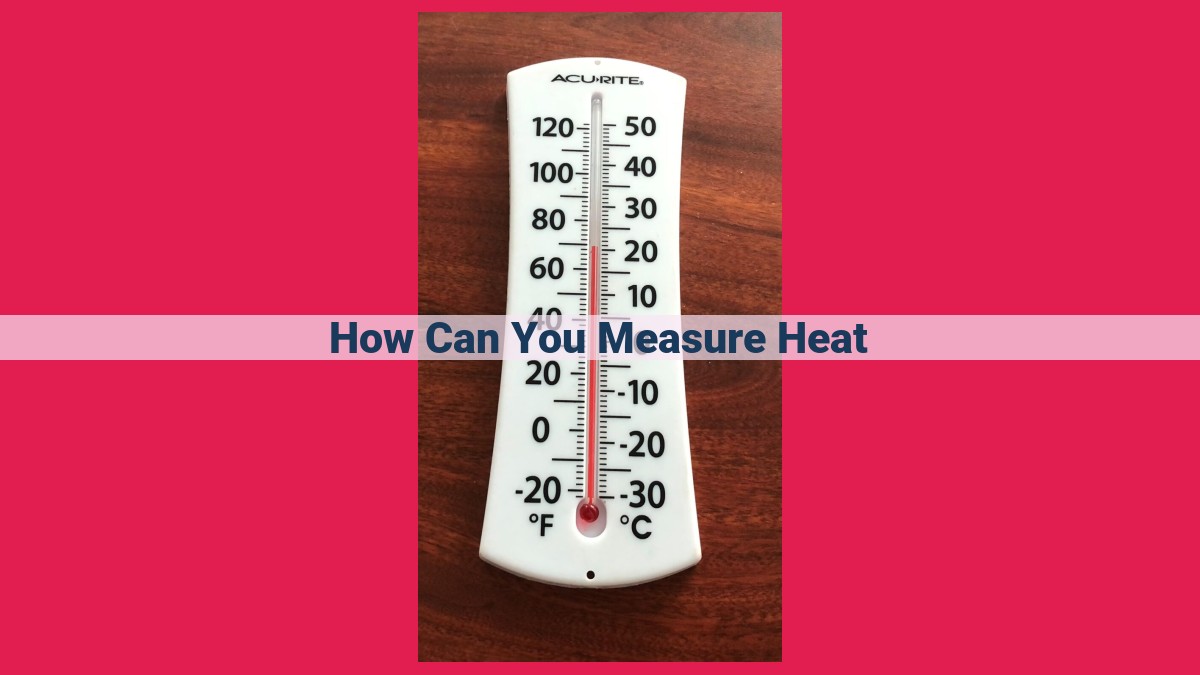
Heat measurement involves determining the amount of thermal energy transferred or absorbed. By understanding specific heat capacity, calorimetry, latent heat, heat transfer mechanisms, and thermal properties like conductivity and temperature gradient, scientists can quantify heat flow using formulas and techniques. Calorimetry measures heat transfer using specific heat capacity, while latent heat considers energy exchange during phase changes. Heat transfer analysis involves conduction, convection, and radiation, influenced by factors like thermal conductivity and surface area. Thermal imaging visualizes heat patterns, aiding in applications such as detecting temperature variations and identifying heat sources.
Understanding Heat Measurement: Specific Heat Capacity
- Define specific heat capacity and its significance in measuring temperature changes.
- Discuss the relationship between specific heat capacity, calorimetry, latent heat, and heat transfer.
Understanding Heat Measurement: The Importance of Specific Heat Capacity
In the world of heat, understanding specific heat capacity is crucial. It’s like a substance’s fingerprint, measuring its ability to resist temperature changes when heated. The higher the specific heat capacity, the harder it is for a substance to warm up.
This property has significant implications in our daily lives. Think about it: When you cook a meal, ingredients with high specific heat capacities, like water, take longer to boil or cool compared to those with lower capacities, like oil. So, if you’re trying to cool down a hot drink, adding ice cubes with their high specific heat capacity will help absorb heat more effectively.
Specific heat capacity also plays a vital role in understanding calorimetry, the science of measuring heat transfer. It allows us to calculate how much heat is transferred between substances when they come into contact with each other. Moreover, it’s closely related to latent heat, which measures the energy required to change a substance’s phase, such as from liquid to gas.
By comprehending the concept of specific heat capacity, we can better understand the flow of heat around us. It’s a fundamental tool in thermal engineering, helping us design efficient heating and cooling systems, as well as understand the thermal behavior of various materials.
Calorimetry: Quantifying the Flow of Thermal Energy
In the realm of thermal science, a crucial tool for unraveling the mysteries of heat transfer and temperature changes is calorimetry. This intricate process allows us to meticulously measure the exchange of heat between substances, providing valuable insights into the intricate dance of thermal energy.
Imagine yourself as a culinary chemist, carefully measuring the heat released or absorbed during a chemical reaction. Calorimetry empowers you to quantify this energy exchange, revealing the enthalpy changes that drive these reactions. But beyond the confines of the laboratory, calorimetry finds diverse applications in industries ranging from engineering to medicine.
At its core, calorimetry relies on the fundamental concept of specific heat capacity. This property represents the amount of heat required to raise the temperature of a unit mass of a substance by one degree. By understanding the specific heat capacities of different materials, we can precisely calculate the heat transfer that occurs when they interact.
In practice, calorimetry involves bringing together two substances of known masses and specific heat capacities in a thermally insulated container called a calorimeter. As the substances exchange heat, the temperature of the system changes. By accurately measuring this temperature change, we can determine the amount of heat transferred and unravel the underlying thermal dynamics.
The principles of calorimetry extend far beyond simple heat exchange. By analyzing temperature changes in a closed system, scientists can decipher the enthalpy of chemical reactions, the latent heat of phase changes, and even the thermal conductivity of materials. Calorimetry empowers us to quantify and understand the intricate flow of thermal energy that shapes our world.
Latent Heat: Energy for Phase Changes
Heat is an essential concept in our everyday lives, from cooking food to powering our homes. But what exactly is heat, and how do we measure it? One key aspect of heat measurement is specific heat capacity, which tells us how much heat is required to raise the temperature of a substance by one degree Celsius.
Another important concept is latent heat. Latent means “hidden,” and latent heat refers to the energy that is absorbed or released during a phase change, such as when a solid turns into a liquid or a liquid turns into a gas. This energy is not reflected in the temperature change of the substance, but it is still a very real form of heat.
Specific heat capacity is a measure of how much heat is required to raise the temperature of one gram of a substance by one degree Celsius. Latent heat, on the other hand, is a measure of how much heat is required to change the phase of one gram of a substance without changing its temperature.
The relationship between specific heat capacity and latent heat is that latent heat is the amount of heat that is required to change the phase of a substance, and specific heat capacity is the amount of heat that is required to raise the temperature of a substance by one degree Celsius.
For example, the latent heat of fusion is the amount of heat that is required to melt one gram of a solid into a liquid. The latent heat of vaporization is the amount of heat that is required to vaporize one gram of a liquid into a gas.
Latent heat is a very important concept in many different fields, such as chemistry, physics, and engineering. It is also important in everyday life, such as when we cook food or use a clothes dryer.
Heat Transfer: The Movement of Thermal Energy
- Explain the concept of heat transfer and its mechanisms of conduction, convection, and radiation.
- Describe the factors that influence heat transfer, such as temperature gradient, thermal conductivity, and surface area.
Heat Transfer: The Movement of Thermal Energy
Imagine a cozy campfire on a chilly night. As you warm your hands over the flames, you witness firsthand the phenomenon of heat transfer. Heat energy travels from the hotter fire to your cooler hands, providing you with warmth. This seemingly simple process involves three fundamental mechanisms: conduction, convection, and radiation.
Conduction: The Direct Path
Think of conduction as heat transfer through a direct contact between two objects. Imagine a metal spoon placed in a hot cup of coffee. Heat energy flows from the hotter coffee to the cooler spoon, raising the spoon’s temperature. The rate of conduction depends on the material’s thermal conductivity, a measure of its ability to transfer heat. Metals like copper and aluminum have high thermal conductivity, allowing heat to move quickly through them.
Convection: Fluid Flows for Heat Exchange
In fluids like air or water, heat is transferred by convection. Warm fluid rises due to its lower density, carrying heat upward. As it rises, it cools and sinks back down, creating a continuous cycle. This process is crucial in heating and cooling systems, such as air conditioners and radiators. The surface area of a fluid-filled object plays a significant role in convection efficiency.
Radiation: Heat Across the Void
Unlike conduction and convection, radiation involves heat transfer through electromagnetic waves. Every object emits thermal radiation, but the hotter the object, the greater its intensity. This is why you feel warmth from a fire even when you’re not touching it. Heat transfer by radiation occurs in all directions, making it an efficient way to transfer heat over distances.
Factors that Govern Heat Transfer
Several factors influence the rate of heat transfer:
- Temperature Gradient: The greater the temperature difference between two objects, the faster heat flows.
- Thermal Conductivity: Materials with high thermal conductivity transfer heat more efficiently.
- Surface Area: Objects with larger surface areas provide more room for heat transfer.
Understanding heat transfer is essential in various fields, from heating and cooling our homes to designing efficient manufacturing processes. By harnessing the power of heat transfer, we enhance our comfort, advance technology, and unlock new possibilities in the world of energy.
Thermal Conductivity: The Crucial Factor in Heat Transfer
In the realm of heat transfer, materials play a pivotal role in determining how efficiently thermal energy flows. This ability of materials to conduct heat is quantified by a property known as thermal conductivity. Imagine a material as a highway for heat, where thermal conductivity represents the width and smoothness of the road. The higher the thermal conductivity, the wider and smoother the road, allowing heat to travel faster and more efficiently.
Thermal conductivity is influenced by several factors, including the material’s atomic structure and molecular bonding. Materials with tightly packed atoms and strong bonds, such as metals, generally have high thermal conductivity. In contrast, materials with loosely packed atoms and weaker bonds, like insulators, have low thermal conductivity.
The relationship between thermal conductivity, temperature gradient, and heat transfer rate is governed by Fourier’s law of heat conduction. According to Fourier’s law, the heat transfer rate (Q) through a material is directly proportional to the thermal conductivity (k), the temperature gradient (dT/dx), and the cross-sectional area (A) perpendicular to the heat flow. Mathematically, it can be expressed as:
Q = -kA (dT/dx)
The negative sign indicates that heat flows from regions of higher temperature to regions of lower temperature.
Understanding thermal conductivity is essential in various applications:
-
Building insulation: Materials with low thermal conductivity, such as fiberglass or foam, are used as insulation to minimize heat loss from buildings.
-
Electronic cooling: Thermal conductivity plays a critical role in the design of electronic devices, as it determines how effectively heat can be dissipated from the components.
-
Medical imaging: Thermal imaging devices utilize the principle of thermal conductivity to detect temperature variations on the skin’s surface, providing insights into underlying physiological processes.
Temperature Gradient: A Driving Force for Heat Flow
- Explain the concept of temperature gradient and its role in heat transfer.
- Describe how temperature gradients drive the flow of heat from higher to lower temperatures.
Temperature Gradient: The Driving Force for Heat Flow
In the realm of heat transfer, the concept of temperature gradient reigns supreme as the driving force that propels thermal energy from one point to another. Picture a landscape with towering mountains and tranquil valleys. Just as gravity draws water downhill, a temperature gradient compels heat to cascade from higher to lower temperatures.
Consider two objects placed in contact with each other, one at a hotter temperature and the other at a cooler temperature. The difference in temperature between these two objects creates a temperature gradient. This gradient acts like an invisible bridge, providing a pathway for heat to flow from the hotter object to the cooler one.
The magnitude of the temperature gradient determines the intensity of heat transfer. A steeper gradient, akin to a steep mountain slope, accelerates heat flow, while a gentler gradient, resembling a gentle hillside, slows it down. This relationship highlights the crucial role of temperature gradient in regulating the rate of heat transfer.
As heat flows down the temperature gradient, it dissipates, warming the cooler object and cooling the hotter object. This process continues until the temperature difference between the two objects diminishes, reaching an equilibrium state. In this state, the temperature gradient disappears, and heat transfer ceases.
Understanding temperature gradients is essential in various fields, including thermal engineering, heating and cooling systems, and even everyday life. By harnessing the power of temperature gradients, engineers can design efficient heating and cooling systems that maintain comfortable indoor environments. Scientists can study the heat flow in geological formations to gain insights into Earth’s processes. And in our homes, temperature gradients guide heat from hot stoves to our food, ensuring evenly cooked meals.
Heat Flux: Unveiling the Rate of Heat Transfer
In the realm of heat transfer, heat flux plays a pivotal role in quantifying the rate at which thermal energy traverses a given area. This crucial parameter measures the amount of heat flowing through a material or surface per unit time and surface area.
The formula for calculating heat flux, denoted by the symbol q, is given by:
q = k * (ΔT/Δx)
where:
- k represents the thermal conductivity of the material (a measure of its ability to conduct heat)
- ΔT is the temperature gradient (the difference in temperature between two points)
- Δx is the thickness of the material
This equation highlights the direct proportionality between heat flux and both thermal conductivity and temperature gradient. Higher thermal conductivity and steeper temperature gradients result in greater heat flux, indicating a more rapid transfer of heat.
Understanding heat flux is essential in various applications, including heat exchanger design, thermal management, and building insulation. It helps engineers and scientists quantify the rate at which heat is transferred through different materials and systems. By manipulating thermal conductivity, temperature gradients, and material thickness, it is possible to optimize heat transfer processes.
Thermal Imaging: Unveiling the Invisible
In the realm of heat measurement, thermal imaging stands as a revolutionary tool, providing us with the ability to visualize temperature differences and gain invaluable insights into thermal patterns.
Principles of Thermal Imaging
Thermal imaging operates on the principle that all objects emit infrared radiation, an electromagnetic wave with a wavelength longer than visible light. The intensity of this radiation corresponds to the temperature of the object. Thermal imaging cameras capture this radiation and convert it into a color-coded image, where warmer areas appear brighter and cooler areas appear darker.
Applications in Various Fields
Thermal imaging has found widespread applications in diverse fields:
- Science: Thermal imaging is used to study heat transfer in materials, detect temperature variations in geological formations, and investigate the thermal properties of biological systems.
- Engineering: Engineers utilize thermal imaging to identify thermal inefficiencies in buildings, detect overheating issues in electrical systems, and monitor the performance of industrial machinery.
- Medicine: Thermal imaging is a valuable tool for diagnosing medical conditions such as inflammation, tumors, and vascular disorders. It also aids in surgical planning and monitoring patient recovery.
Revealing Hidden Heat Patterns
Thermal imaging provides a unique perspective that allows us to uncover previously unseen thermal patterns:
- Heat Loss Detection: Thermal imaging helps detect areas of heat loss in buildings, enabling homeowners to improve insulation and reduce energy consumption.
- Electrical Fault Diagnosis: Thermal imaging can pinpoint overheating electrical components, preventing potential fires and ensuring electrical safety.
- Medical Diagnostics: Thermal imaging can differentiate between benign and cancerous growths based on their different thermal signatures. It also helps locate and monitor blood clots and infections.
Thermal imaging has revolutionized the field of heat measurement, providing us with the ability to visualize temperature differences and gain profound insights into thermal patterns. Its applications span a wide range of fields, from science and engineering to medicine, offering invaluable benefits and enhancing our understanding of the invisible world of heat.