The Significance Of ‘Q’ In Scientific And Practical Applications: Understanding Magnitude, Units, And Impacts
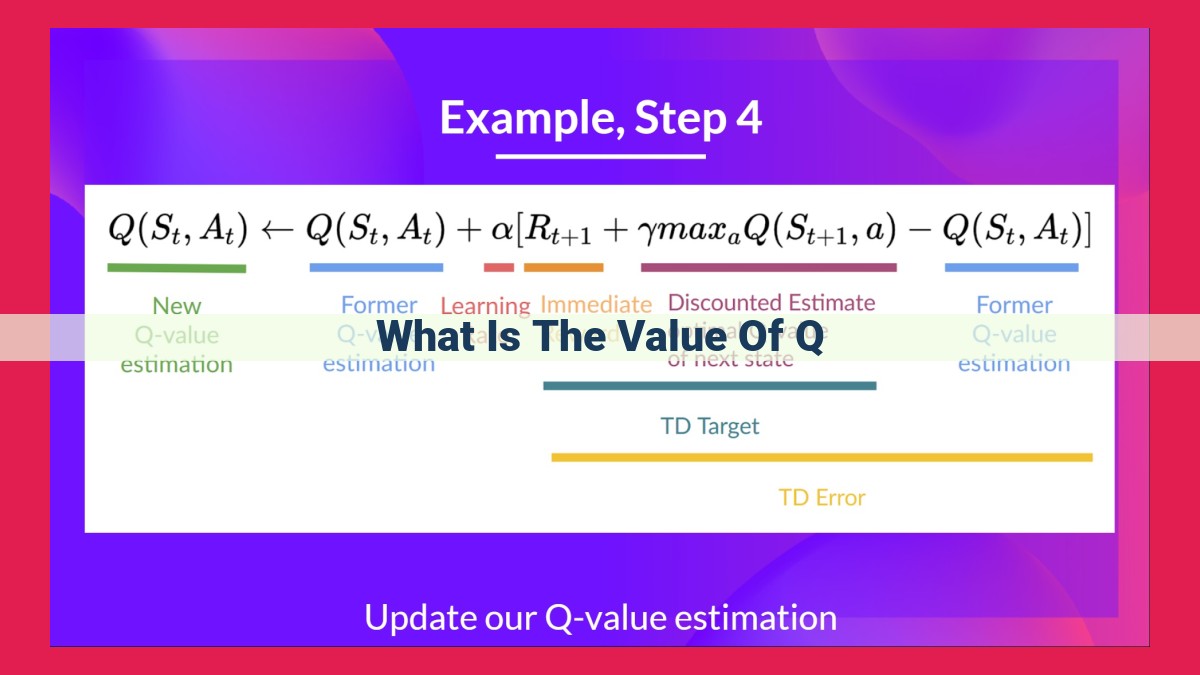
The value of q denotes the magnitude of a variable and often represents a ratio or quotient. Its units of measurement vary depending on the context, but frequently include dimensionless quantities or units derived from fundamental physical constants. Q can range from minimum to maximum values within an established range, influenced by other variables. Its variation affects various outcomes or phenomena, making it an important factor in scientific and practical applications across diverse fields.
Unraveling the Enigmatic World of q
q, an enigmatic entity in the realm of science, holds immense significance, influencing a myriad of phenomena. Its magnitude and numerical value are intricate concepts that we shall delve into, unveiling the secrets it cloaks.
At its core, q represents a dimensionless quantity, devoid of units. It manifests as a ratio, a comparison between two distinct values, often representing the relationship between two variables. In this sense, q serves as a multiplier, scaling one variable relative to the other.
The numerical value of q can vary infinitely, spanning the entire number line. It can assume positive or negative values, catering to a wide range of scenarios. However, in specific contexts, q may be constrained within a particular range, governed by the underlying physical or mathematical principles.
Comprehending the magnitude and numerical value of q is paramount to unlocking its true potential. By understanding these fundamental aspects, we empower ourselves to interpret its impact and harness its power in unraveling the mysteries of the universe.
Units of Measurement for q: Unraveling the Language of Quantifiable Value
In the realm of science and engineering, understanding the units of measurement used for various quantities is crucial for accurate communication and consistent interpretation. Just as money has units like dollars or euros, quantifiable values like q require specific units to express their magnitude and significance.
The units of q serve as a universal language, enabling scientists and researchers across the globe to compare, analyze, and build upon each other’s work. They provide a standardized reference point, ensuring that everyone is on the same page when discussing the value of q.
The SI (International System of Units), an internationally recognized system of measurement units, establishes the standard units for q. Depending on the context and specific field of study, q may be expressed in different SI units. These units are carefully chosen to reflect the nature of q and its relationship with other physical quantities.
Understanding the units of measurement used for q is not just a matter of technicality; it’s about ensuring precision and clarity in scientific discourse. It allows us to communicate complex ideas effectively, avoid misunderstandings, and build a solid foundation for scientific progress.
The Range of q: Exploring its Boundaries
In the realm of physics and mathematics, the enigmatic quantity known as q often plays a pivotal role. Understanding its range – the boundaries within which its values can fluctuate – is crucial for comprehending its significance and the phenomena it governs.
The minimum value of q is generally determined by the specific context in which it is encountered. In certain situations, q may be constrained to be non-negative, while in others, it may be allowed to assume both positive and negative values. The maximum value of q, on the other hand, is often set by physical or mathematical limitations. Beyond this threshold, the behavior of q may become undefined or inconsistent with the theoretical framework.
Defining the valid range of q is essential for its meaningful interpretation. This range encompasses all permissible values that q can adopt without violating any fundamental principles or constraints. Establishing the valid range of q helps researchers, engineers, and other professionals to draw meaningful conclusions and make informed decisions based on its numerical value.
Understanding the range of q is a key step towards unlocking its full potential and unraveling its secrets. By comprehending its boundaries, we can better appreciate the role it plays in shaping the universe we inhabit and the technologies we rely upon.
Dependence of q on Other Variables
Every story has its characters and a plot, and in the world of physics, q is a character that plays a pivotal role. Just like our actions are influenced by the people around us, q is also swayed by a cast of supporting variables. So, let’s embark on a journey to uncover the secrets behind q‘s dependence.
The Variable Ensemble
Just as a chess game has its pawns, knights, and queens, q is influenced by a group of variables that act like its companions. These variables, like x, y, and z, are the driving forces that shape q‘s destiny. They can be positive or negative, pulling q in different directions.
Understanding the Interplay
The relationship between q and its variable crew is a delicate dance. Imagine q as a boat sailing on a vast ocean, where x, y, and z represent the currents and winds that push and pull it. When x increases, it might create a favorable current, propelling q towards higher values. On the contrary, a decrease in y could act like a headwind, slowing q‘s progress.
Factors Governing q’s Fate
The influence of x, y, and z on q is not merely a game of chance. It is governed by certain factors, the hidden rules of the universe. These factors determine the strength and direction of the forces that act upon q. For instance, the relationship between q and x might be linear, meaning that a proportional change in x leads to a predictable change in q.
Implications of Variable Influence
Understanding the dependence of q on other variables is not just an academic exercise. It has real-world implications. Engineers and scientists rely on this knowledge to design systems and predict outcomes. By manipulating q‘s companions, they can control its behavior and achieve desired results.
The journey to unravel the secrets of q has led us to the realization that its value is not set in stone. Instead, it dances to the tune of a supporting cast of variables and factors. By understanding this dynamic interplay, we gain the power to predict q‘s behavior and harness its potential in the world of science and engineering.
The Profound Effects of Varying q
In the realm of physics, the enigmatic parameter known as q holds sway over a multitude of phenomena. Its value, whether minuscule or astronomical, wields the power to transform the outcome of countless interactions.
When q diminishes, a subtle dance ensues. Systems become more stable, resisting change with an unwavering resolve. Like a ship anchored in a calm harbor, their motion is quelled, ensuring a tranquil existence. Conversely, as q ascends, a surge of instability sweeps through the system. It becomes a tempestuous sea, where unpredictability reigns supreme. Particles ignite with renewed vigor, clashing against one another in a chaotic symphony.
The impact of varying q extends beyond the confines of theoretical models. In the realm of electronic circuits, its manipulation allows us to control the flow of current and shape the behavior of transistors. Like a maestro orchestrating an electrical symphony, engineers exploit q to create devices that power our modern world.
In the enigmatic world of quantum mechanics, q takes center stage, defining the enigmatic realm of superposition. By tweaking its value, we can coax particles into occupying multiple states simultaneously, creating a paradoxical dance between reality and possibility. It is in these quantum playgrounds that we glimpse the true nature of our enigmatic universe.
Thus, the seemingly innocuous parameter q emerges as a transformative force, its value dictating the behavior of systems across vast domains. From the stability of matter to the enigmatic realm of quantum mechanics, q weaves its influence, shaping the tapestry of our existence.
Applications of q
The value of q, a versatile parameter, extends beyond theoretical considerations into a myriad of practical applications across diverse fields. Its significance lies in its ability to quantify and predict real-world phenomena.
In the realm of engineering, q plays a crucial role in designing and optimizing structures. By meticulously calculating the thermal conductivity of building materials, engineers can ensure efficient insulation and minimize energy loss. Precisely determining the electrical conductivity of materials is paramount for optimizing electronic devices and ensuring their reliable performance.
Within the medical domain, q finds its application in diagnostic imaging. By analyzing the magnetic susceptibility of tissues using MRI scanners, physicians can differentiate between healthy and diseased cells. Additionally, q is pivotal in radiation therapy, where its accurate measurement enables precise targeting of tumors and minimizes damage to surrounding tissues.
In the manufacturing industry, q serves as a key parameter in controlling the quality of products. By monitoring the flow rate of liquids and gases in production processes, manufacturers can ensure consistency and prevent defects. Moreover, q plays a vital role in optimizing manufacturing processes by regulating temperature and pressure.
In agriculture, q is an essential tool for evaluating soil fertility. By measuring soil moisture content, farmers can determine optimal irrigation schedules, preventing crop damage and maximizing yields. Additionally, q is used in studying plant growth and predicting crop yield.
These diverse applications underscore the practical importance of q in various fields. Its precise measurement and analysis empower professionals to solve complex problems, optimize processes, and advance human knowledge.