Plasma: Applications, Generation Methods, Advantages, And Disadvantages
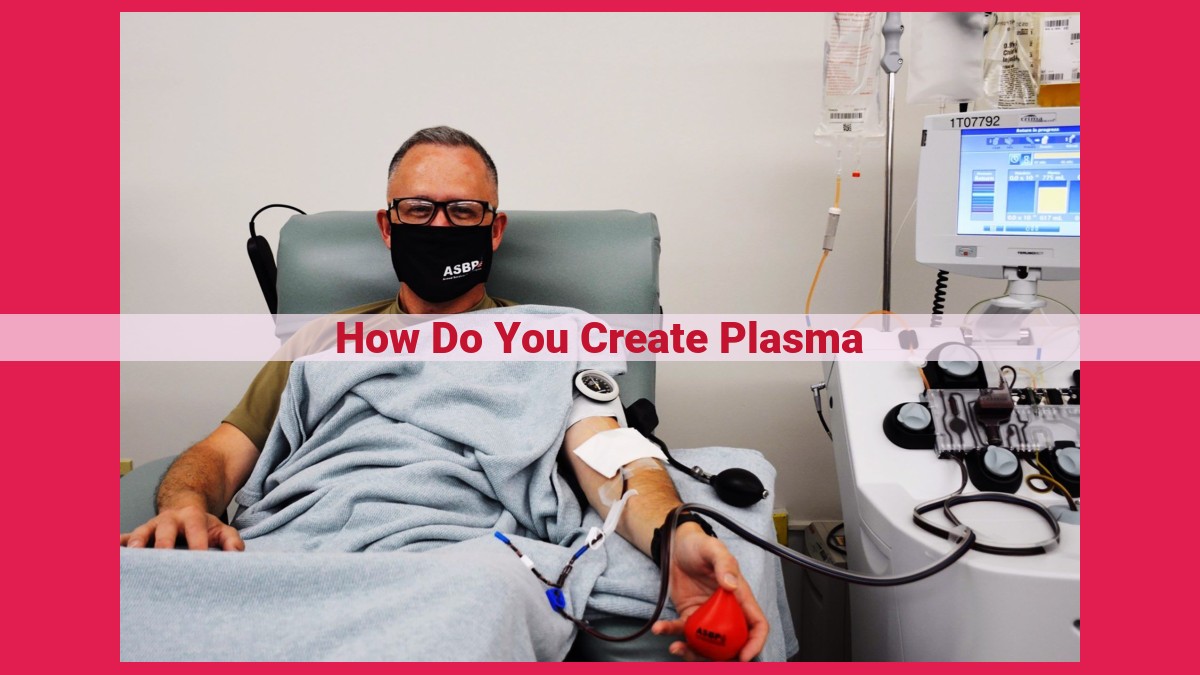
Plasma, an ionized gas, is created by ionizing the gas through various processes: thermal, collisional, photoionization, electric/magnetic field ionization. Plasma generation methods include laser-induced, microwave-induced, radiofrequency, and DC/AC plasma. Plasma finds applications in material processing, semiconductor manufacturing, aerospace propulsion, medical devices, and scientific research. However, it has advantages and disadvantages, such as high energy density, low conductivity, and temperature-dependent reactivity.
Unraveling the Enigmatic World of Plasma
Plasma, the fourth state of matter, is an ionized gas that shares properties with both gases and liquids. Unlike gases, it is electrically conductive and responds to magnetic fields. These unique characteristics stem from the fact that plasma particles, ions, and electrons are separated from each other, creating a charged sea of energy.
The energy required to ionize a gas depends on its electron configuration and temperature. When the temperature of a gas is raised, electrons gain sufficient energy to break free from their atomic bonds, forming ions. This process, known as thermal ionization, is commonly used in plasma generation.
Other methods of ionization exist, such as collisional ionization where high-energy electrons collide with gas molecules, photoionization where photons interact with gas atoms, electric field ionization where an applied electric field pulls electrons away from atoms, and magnetic field ionization where a magnetic field compresses electrons.
Understanding plasma’s unique properties and the processes involved in its generation opens the door to unlocking its immense potential. Plasma finds applications in diverse fields, ranging from material processing and semiconductor manufacturing to aerospace propulsion and medical advancements. This transformative state of matter holds the key to countless innovations and continues to captivate scientists and engineers alike.
Ionization Processes: The Birth of Plasma
In the realm of science, plasma reigns supreme as the most abundant state of matter in the universe. To understand this enigmatic substance, we must delve into the fascinating world of ionization processes, the very mechanisms that transform ordinary gases into the extraordinary plasma.
Thermal Ionization: A Heated Affair
Imagine a fervent debate escalating in a heated room where temperatures soar. The atmosphere grows tense, and sparks begin to fly. In this metaphorical realm, thermal ionization takes place. When a gas is subjected to extreme temperatures, typically above 10,000 degrees Celsius, the intense heat strips electrons from atoms, leaving behind positively charged ions.
Collisional Ionization: A Forceful Encounter
Picture a high-speed car race, where collisions are not mere accidents but deliberate collisions. In the world of ionization, collisional ionization occurs when energetic electrons or ions bombard neutral gas particles. The impact is so forceful that it ejects electrons from their atomic orbits, creating new ions.
Photoionization: Light’s Healing Touch
In the realm of ionization, there exists a phenomenon akin to photosynthesis. Photoionization harnesses the power of light, specifically ultraviolet or X-ray radiation. When these high-energy photons interact with gas atoms, they impart enough energy to liberate electrons, resulting in ionization.
Electric Field Ionization: A Field of Force
Imagine a battlefield where electric fields are the generals, commanding charged particles to do their bidding. In electric field ionization, a strong electric field accelerates free electrons within a gas. As they gain momentum, they collide with neutral atoms, transferring their energy and ionizing them in the process.
Magnetic Field Ionization: A Twist of Fate
In the realm of electromagnetism, there exists an alternative path to ionization. Magnetic field ionization utilizes the power of magnetic fields to trap electrons within a gas. Over time, these trapped electrons accumulate enough energy through collisions to ionize neutral atoms and create plasma.
Plasma Generation Methods
Plasma, a unique state of matter, can be artificially created through various methods. These techniques harness different energy sources to ionize gases, transforming them into electrically charged plasmas.
Laser-induced Plasma (LIP)
LIP employs a high-intensity laser to vaporize and ionize a sample. The laser’s focused beam creates a localized plasma, which can be employed for spectrochemical analysis and material modification.
Microwave-induced Plasma (MIP)
MIP utilizes microwave energy to excite and ionize gases. This method is commonly used in analytical chemistry for atomic emission spectroscopy and mass spectrometry applications.
Radiofrequency Plasma (RFP)
RFP generates plasma using radiofrequency energy. The oscillating electric field ionizes gas molecules, creating a sustained plasma source. RFP finds applications in semiconductor processing, surface treatment, and plasma etching.
DC Plasma
DC plasma is created by applying a direct current (DC) voltage to electrodes placed in a gas. The electric field accelerates electrons, which collide with gas molecules, leading to ionization. This method is used in plasma displays, fluorescent lighting, and medical devices.
AC Plasma
AC plasma utilizes an alternating current (AC) voltage to generate plasma. The changing electric field causes electrons to oscillate, leading to ionization. AC plasma is used in plasma cutters, welders, and high-power lasers.
Pulsed Plasma
Pulsed plasma is generated by applying short, high-power pulses of energy to a gas. This method creates a transient plasma that can be used for pulsed lasers, electromagnetic propulsion, and medical applications.
Continuous Plasma
Continuous plasma is sustained without the need for pulsed energy input. This method utilizes a continuous energy source, such as microwaves or radiofrequency, to maintain a stable plasma. Continuous plasma is used in plasma torches, plasma reactors, and fusion energy research.
The choice of plasma generation method depends on the specific application and the desired plasma properties, such as temperature, density, and stability. These techniques provide a versatile means to create and harness the unique properties of plasma for various scientific, industrial, and medical applications.
Applications of Plasma: A Versatile Material with Diverse Uses
Plasma, the fourth state of matter, offers a unique set of properties that make it incredibly versatile in a wide range of applications across various industries. Its high energy density and reactivity allow it to perform tasks that are impossible for solids, liquids, or gases.
Material Processing
Plasma is extensively used in material processing due to its ability to modify the surface properties of materials. Plasma etching uses plasma to remove material from a substrate, creating precise patterns and structures used in semiconductor manufacturing and microelectronics. Plasma deposition involves using plasma to coat surfaces with thin films, improving their properties for wear resistance, corrosion protection, and electrical insulation.
Semiconductor Manufacturing
Plasma plays a crucial role in the fabrication of semiconductors, the building blocks of modern electronics. Plasma etching is used to create the intricate patterns on silicon wafers, while plasma deposition is employed to create thin insulating layers between transistors. The use of plasma ensures precise control over the dimensions and properties of these semiconductor devices.
Aerospace Propulsion
Plasma finds application in aerospace propulsion. Plasma engines generate thrust by accelerating plasma, offering high specific impulse and efficiency for spacecraft propulsion. This technology is particularly valuable for interplanetary travel and deep space exploration.
Medical Devices
Plasma has made significant contributions to the medical field. Plasma scalpels use focused plasma to precisely cut tissue during surgeries, reducing bleeding and scarring. Plasma sterilization utilizes plasma to eliminate bacteria and viruses from medical instruments and surfaces, enhancing patient safety.
Scientific Research
Plasma is a valuable tool for scientific research. Plasma diagnostics helps scientists study the behavior of plasma in various environments, such as fusion reactors and astrophysical plasmas. Plasma accelerators generate high-energy beams of ions for particle physics research and cancer therapy.
In summary, plasma’s unique properties make it a highly versatile material with applications ranging from material processing to scientific research. Its ability to modify surfaces, create thin films, generate thrust, sterilize medical devices, and advance scientific understanding has made plasma an indispensable tool in various industries, shaping our world in countless ways.
Advantages and Disadvantages of Plasma
Plasma, the fourth state of matter, holds immense potential and complexity. While it offers unique advantages, it also presents challenges that must be carefully considered.
Advantages:
-
High Energy Density: Plasma possesses an extremely high energy density, making it an efficient source of power. This energy can be harnessed for various applications, including nuclear fusion and advanced propulsion systems.
-
Low Conductivity: Despite its high energy content, plasma exhibits low electrical conductivity. This property allows for precise control and manipulation of the plasma in various devices and processes.
-
Reactivity at Different Temperatures: Plasma can react with different substances at widely varying temperatures. This adaptability makes it suitable for a diverse range of applications, from surface modification to medical therapies.
Disadvantages:
-
Low Conductivity: The low electrical conductivity of plasma can also be a disadvantage. It limits the ability to transmit high currents through plasma and can pose challenges in creating stable and efficient plasma discharges.
-
Reactivity: While the reactivity of plasma can be advantageous, it can also lead to unintended reactions and material damage. Careful control and containment of plasma are crucial to minimize these risks.
-
High Temperature: Plasma often operates at extremely high temperatures. This requires the use of specialized materials and cooling systems to prevent equipment damage and ensure safe operation.
In summary, plasma offers a range of advantages, including high energy density, low conductivity, and reactivity at different temperatures. However, it also presents challenges related to low conductivity, reactivity, and high temperatures. By carefully considering these factors, researchers and engineers can harness the power of plasma while mitigating its potential drawbacks.