Optimize Photosynthesis For Enhanced Plant Growth And Productivity: Understanding Limiting Factors
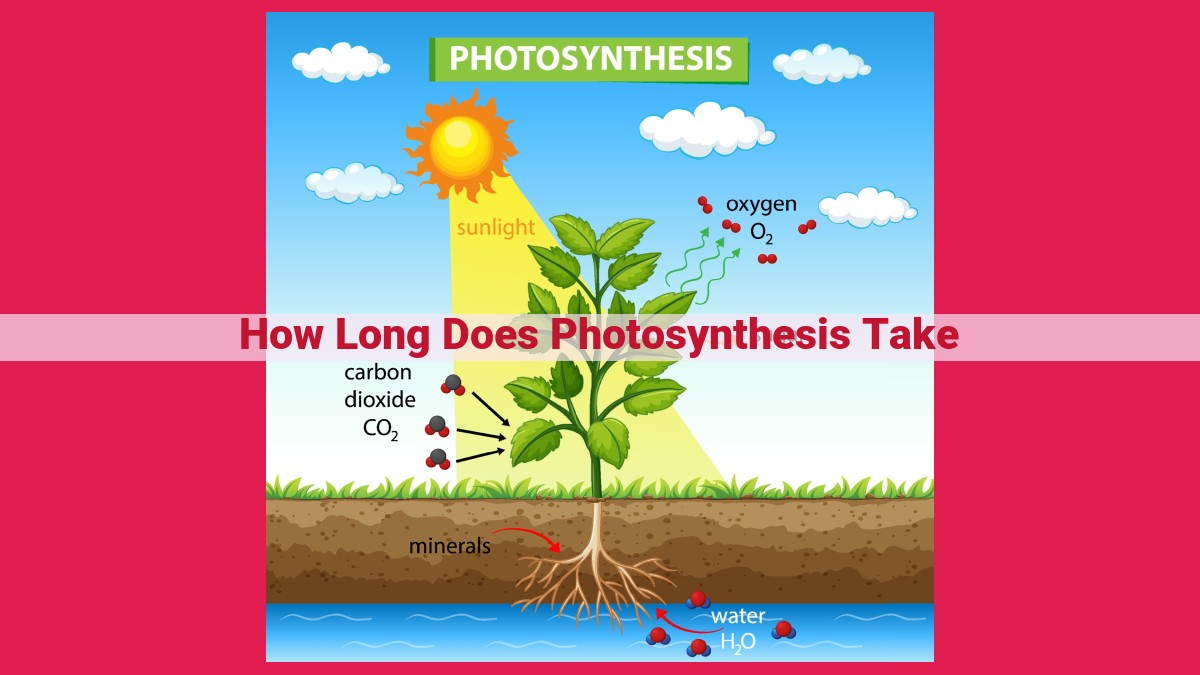
The rate of photosynthesis is influenced by various factors that determine the time it takes to complete the process. These factors include the rate-limiting steps in the process, light intensity, temperature, CO2 concentration, and leaf thickness. Understanding these factors is crucial for optimizing photosynthesis efficiency and enhancing plant growth and productivity.
Photosynthesis: Unveiling the Timeline of Life’s Essential Process
Embark on a journey to understand the intricate dance of photosynthesis, the process that sustains life on Earth. By delving into the factors that govern its pace, we unlock the secrets of plant growth and productivity.
The Rate-Limiting Step: The Bottleneck of Photosynthesis
Imagine a production line, where each step is crucial for the final product. Photosynthesis operates similarly, with a cascade of reactions, each with its own pace. The slowest of these steps, known as the rate-limiting step, determines the overall speed of photosynthesis.
Factors such as enzyme activity can influence the rate-limiting step. Enzymes are the catalysts that accelerate reactions, and their abundance or effectiveness can affect the pace of photosynthesis. Substrate concentration, the availability of raw materials for the reaction, also plays a role. Additionally, environmental conditions like temperature and pH can alter enzyme activity and substrate availability, impacting the rate-limiting step and, thus, the overall process.
Light Intensity’s Impact on Photosynthesis
Light is the driving force behind photosynthesis, the process that converts sunlight into energy for plants. Understanding the relationship between light intensity and the rate of photosynthesis is crucial for optimizing plant growth and productivity.
Light-Dependent Reactions
Photosynthesis occurs in two stages: light-dependent reactions and light-independent reactions (also known as the Calvin cycle). Light-dependent reactions take place in the thylakoid membranes of chloroplasts and require light energy to produce oxygen, generate energy carriers (ATP and NADPH), and split water molecules.
Light Intensity and Photosynthesis Rate
The intensity of light dramatically influences the rate of photosynthesis. As light intensity increases, the rate of photosynthesis generally increases until it reaches a plateau. This is known as light saturation. At this point, the photosynthetic machinery is operating at maximum capacity, and any further increase in light intensity does not result in a significant increase in the rate of photosynthesis.
Light Saturation
The light saturation point varies among plant species and environmental conditions. In general, sun-loving plants, such as sunflowers and tomatoes, have higher light saturation points compared to shade-loving plants, such as ferns and mosses. This adaptation allows sun-loving plants to thrive in high-light environments, while shade-loving plants can survive in lower-light conditions.
Implications for Plant Growth
Understanding the role of light intensity in photosynthesis is essential for managing plant growth and productivity. Insufficient light can limit plant growth, reduce yields, and increase susceptibility to pests and diseases. On the other hand, excessive light can lead to photoinhibition, a process that damages the photosynthetic apparatus and reduces plant productivity.
By manipulating light intensity through artificial lighting or canopy management techniques, it is possible to optimize photosynthesis and enhance plant growth in controlled environments and agricultural settings.
The Impact of Temperature on Photosynthesis: A Tale of Enzyme Activity and Root Respiration
Photosynthesis, the life-giving process that converts sunlight into energy for plants, is a complex dance of molecular interactions. One of the key players in this intricate choreography is temperature, a silent maestro that influences the rhythm and efficiency of the photosynthetic process.
Enzyme Activity: The Dancers’ Grace
Enzymes, the tiny protein molecules responsible for facilitating chemical reactions, are highly sensitive to temperature. As the temperature rises, enzyme activity initially increases, allowing for a faster pace of photosynthesis. However, beyond an optimal temperature, enzymes begin to deteriorate and lose their effectiveness, leading to a slowdown in the photosynthetic process.
Soil Temperature: The Roots’ Contribution
While the air temperature directly affects leaf metabolism, the soil temperature plays an equally crucial role in photosynthesis. Cooler soil temperatures reduce root respiration, the process by which roots consume energy for nutrient uptake. This lower energy demand allows more energy to be diverted towards nutrient absorption, providing essential building blocks for photosynthesis.
Diurnal Temperature Range and Frost: The Chilly Challenges
Plants have evolved to adapt to their specific temperature ranges. However, extreme temperature fluctuations can disrupt photosynthesis. A large diurnal temperature range can stress plants, leading to slower growth and reduced photosynthetic efficiency. Similarly, frost can damage plant tissues, reducing their ability to absorb sunlight and carry out photosynthesis.
Temperature is a critical factor that shapes the timeline of photosynthesis. It influences enzyme activity, root respiration, and plant resilience to temperature fluctuations. Understanding the relationship between temperature and photosynthesis allows us to manipulate these conditions to optimize plant growth and productivity. By providing ideal temperatures, we can accelerate photosynthesis, unlocking the full potential of plants to sustain life on Earth.
CO2 Concentration: A Crucial Factor in Photosynthesis
Carbon dioxide (CO2) plays a vital role in photosynthesis, the process by which plants convert sunlight into energy. As a reactant in this process, CO2 is indispensable for the production of sugars, carbohydrates, and other essential compounds.
Several factors influence the availability of CO2 for photosynthesis. Atmospheric CO2 levels have a significant impact. As the concentration of CO2 in the atmosphere increases, the rate of photosynthesis also increases, up to a certain point. This is why plants tend to thrive in areas with higher atmospheric CO2 levels.
Soil CO2 availability also plays a crucial role. Carbon dioxide is released into the soil through microbial respiration and root respiration. Soil factors like moisture, temperature, and pH influence the production and release of CO2, thereby affecting plant growth.
Stomatal conductance, the opening and closing of pores on leaves, regulates the exchange of CO2 and water vapor. Optimal stomatal conductance allows for sufficient CO2 uptake while minimizing water loss. Factors such as light intensity, humidity, and plant water status can influence stomatal conductance.
By understanding the influence of CO2 concentration on photosynthesis, we can explore ways to optimize this process for enhanced plant growth and productivity. Manipulating atmospheric CO2 levels, improving soil conditions, and regulating stomatal conductance through genetic engineering or environmental management techniques offer potential avenues for increasing photosynthesis efficiency.
Leaf Thickness: A Vital Factor in Photosynthesis
As we delve deeper into the intricacies of photosynthesis, it becomes evident that leaf thickness plays a crucial role in this life-sustaining process. Leaf thickness not only influences the absorption of light, but also affects the internal diffusion of carbon dioxide (CO2), which is a vital reactant in photosynthesis.
Impact on Light Absorption
Leaves with greater thickness possess a higher capacity to capture sunlight. This is because thicker leaves contain more chloroplasts, the organelles responsible for capturing light energy and initiating photosynthesis. The increased surface area of thicker leaves allows for efficient light interception, maximizing the leaf’s ability to harness solar energy.
Internal CO2 Diffusion
Leaf thickness also impacts the diffusion of CO2 into the leaf’s interior. CO2 is absorbed through stomata, tiny pores on the leaf surface. However, thicker leaves have fewer stomata per unit area compared to thinner leaves. This can potentially limit the availability of CO2 for photosynthesis, especially when the stomata are closed during water stress or high temperatures.
Determining Factors of Leaf Thickness
Several factors contribute to leaf thickness, including:
- Leaf area index (LAI): The total leaf area per unit ground area
- Specific leaf area (SLA): The leaf area per unit leaf mass
- Leaf mass per unit area (LMA): The leaf mass per unit leaf area
- Cuticle thickness: The waxy layer on the leaf surface
Implications for Photosynthesis
Understanding the impact of leaf thickness on photosynthesis has significant implications for optimizing plant growth and productivity. By manipulating leaf thickness through genetic engineering or cultural practices, scientists can potentially enhance photosynthetic efficiency and improve plant yields. For instance, increasing leaf thickness can increase light absorption and CO2 diffusion, leading to higher rates of photosynthesis.
In summary, leaf thickness is a critical factor in photosynthesis, influencing both light absorption and internal CO2 diffusion. Understanding the role of leaf thickness allows us to develop strategies to manipulate this trait and harness its potential for enhancing plant productivity.