Organic Molecules: Properties, Behaviors, And Applications Defined By Structural Features
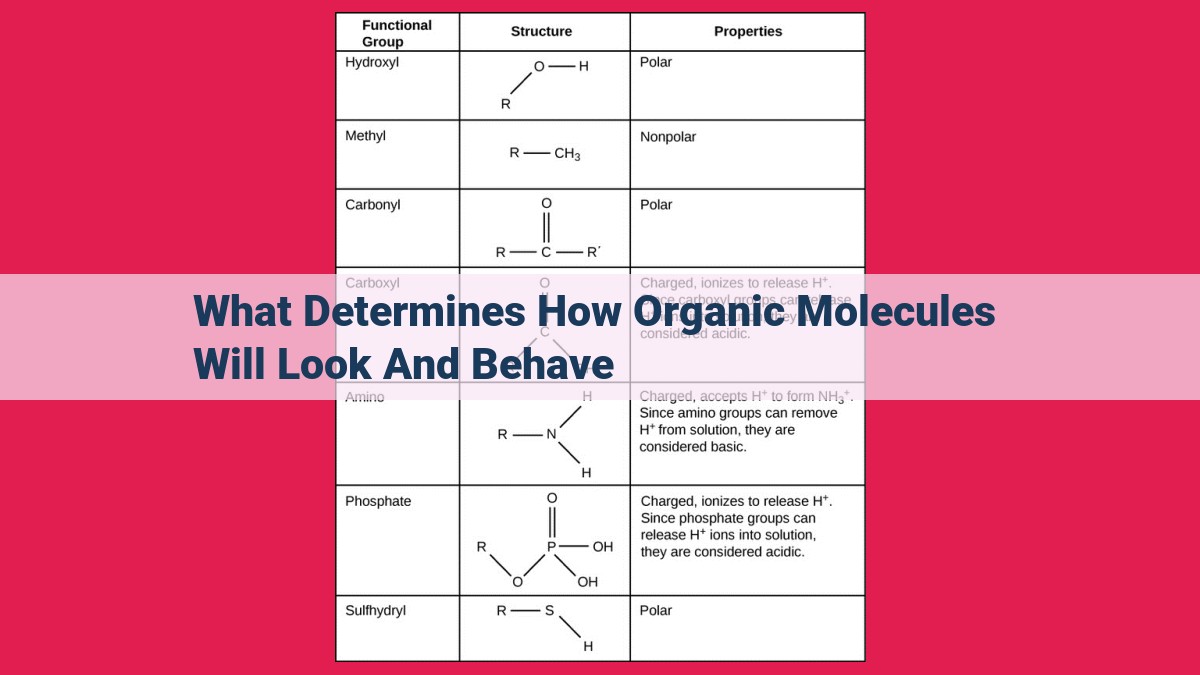
Organic molecules exhibit varying characteristics and behaviors due to their unique molecular structures. Functional groups, polarity, shape, size, and hybridization determine these properties. Functional groups, composed of specific atomic combinations, dictate chemical reactivity. Molecular weight influences physical properties like boiling point and density. Polarity, which arises from uneven charge distribution, affects solubility and intermolecular interactions. Shape, determined by bond angles, impacts molecular organization and reactivity. Size influences solubility and membrane permeability. Hybridization, the mixing of atomic orbitals, determines bond strength, molecular geometry, and reactivity. Understanding these factors enables chemists to predict and modify the properties of organic molecules for various applications in medicine, materials science, and many other fields.
The Fascinating World of Molecular Structure: Unveiling the Essence of Organic Molecules
In the realm of chemistry, organic molecules hold a captivating presence, playing a crucial role in the symphony of life. Their remarkable properties and diverse applications stem from their intricate molecular structure, the blueprint that defines their chemical behavior and biological significance.
Molecular structure is the arrangement of atoms, bonds, and functional groups within a molecule. It’s the foundation upon which all other properties emerge, shaping everything from an organic molecule’s physical appearance to its chemical reactivity. Understanding molecular structure is akin to deciphering the secrets of a molecule’s identity and purpose.
The significance of molecular structure extends far beyond the realm of the laboratory. It underpins the functionality of countless materials we encounter daily, from pharmaceuticals to food additives, plastics, and fragrances. It guides the design of new drugs and therapies, enables the development of sustainable energy sources, and paves the way for innovative materials with extraordinary properties.
Exploring the intricate tapestry of molecular structure is an adventure into the heart of chemistry, unveiling the fundamental principles that govern the behavior of matter. Join us as we delve into the fascinating world of molecular structure, unraveling its secrets and discovering its profound impact on our lives.
Unveiling the Properties of Organic Molecules: A Comprehensive Guide
Let’s embark on an exciting journey to understand the intricate world of organic molecules. These molecules are the building blocks of life and countless materials around us. By delving into their properties, we can unlock their secrets and appreciate the elegance of their design.
Molecular Structure: The Foundation
Every organic molecule has a unique molecular structure, a spatial arrangement of its constituent atoms. This structure governs the molecule’s properties and behavior. Key concepts like functional groups (specific regions with characteristic reactivity), polarity (uneven distribution of electrical charge), and shape play crucial roles in determining these properties.
Functional Groups: The Building Blocks
Functional groups are groups of atoms that confer specific chemical characteristics to organic molecules. They are the reactive centers that participate in chemical reactions and determine a molecule’s polarity, molecular weight, and other traits.
Molecular Weight: Measuring Mass
Molecular weight measures the mass of an organic molecule relative to the mass of a single carbon atom. It provides insights into the size and complexity of the molecule, influencing its solubility, acidity, and other properties.
Polarity: The Dance of Charges
Polarity arises when different atoms in a molecule have unequal electronegativities, leading to a separation of electrical charge. This polarity affects molecular attraction, solubility, and biological interactions.
Shape: The Three-Dimensional Architecture
Molecular shape refers to the three-dimensional arrangement of atoms in a molecule. It is determined by bond angles, connectivity, and the presence of functional groups. Shape can influence a molecule’s reactivity, solubility, and optical activity.
Functional Groups: The Chemical Architects of Organic Molecules
In the realm of organic chemistry, functional groups reign supreme as the architects of chemical properties. Think of them as the master builders that determine the unique characteristics of every organic molecule. These molecular adornments, with their varying shapes and sizes, bring life to the building blocks of organic chemistry.
Unveiling the Power of Functional Groups
Functional groups are specific arrangements of atoms within an organic molecule that dictate its chemical reactivity and physical properties. They are akin to molecular flags, signaling their presence and orchestrating the molecule’s behavior.
Consider the polar hydroxyl group (-OH), found in alcohols and phenols. Its electronegative oxygen atom creates a partial negative charge, making it a ‘thirsty’ proton acceptor and giving alcohols their characteristic acidic nature.
In contrast, the nonpolar hydrocarbon group (-CH3), found in alkanes, is a veritable introvert. Its electrons are evenly distributed, resulting in a neutral charge and a reluctance to participate in chemical reactions.
The Symphony of Molecular Properties
The presence of functional groups not only influences chemical reactivity but also casts a spell on other molecular properties:
-
Molecular Weight: Functional groups contribute to the overall molecular weight, with heavier groups like halogens or nitro groups increasing the mass.
-
Polarity: Polar functional groups, such as alcohols or ketones, create dipoles within the molecule, making it polar overall.
-
Shape: Functional groups can alter the geometry of a molecule, affecting its shape and influencing its physical properties like boiling point or solubility.
-
Solubility: Polar functional groups improve solubility in polar solvents, while nonpolar groups favor nonpolar solvents.
-
Acidity and Basicity: Functional groups can make a molecule acidic or basic by stabilizing or destabilizing ions. For example, carboxylic acids (-COOH) donate protons easily, making them acidic.
-
Optical Activity: Chiral functional groups can give a molecule optical activity, the ability to rotate plane-polarized light. This property is crucial in pharmaceutical and biological applications.
Functional groups are the very essence of organic molecules, imbuing them with a rich tapestry of chemical properties. By understanding the role of functional groups, chemists can predict reactivity, design new compounds, and unravel the intricacies of life’s molecular tapestry.
Functional Groups: The Gatekeepers of Chemical Identity
In the captivating realm of organic molecules, functional groups emerge as indispensable gatekeepers that dictate their chemical personalities. These alluring units, adorned with specific atoms and their connecting webs, play a pivotal role in determining the properties and behaviors of these molecular inhabitants.
Molecular Structure: The Architectural Blueprint
The intricate tapestry of molecular structure weaves the very essence of organic molecules. It orchestrates the precise arrangement of atoms and bonds, forming a three-dimensional canvas upon which chemical interactions unfold. This intricate blueprint influences the strategic placement of functional groups, akin to an architect meticulously planning the distribution of rooms in a house.
Polarity: The Dance of Attraction and Repulsion
Polarity emerges from the dance of electronegativity, the inherent attraction of atoms for electrons. Within functional groups, this dance generates an asymmetry of charge, creating a molecular dipole. Polarity shapes the solubility, reactivity, and other fundamental characteristics of organic molecules.
Molecular Weight: A Measure of Substance
Molecular weight, measured in atomic mass units (amu), reflects the cumulative mass of all the atoms strung together within a molecule. This weighty characteristic correlates with several molecular properties, such as density, boiling point, and vapor pressure.
Hybridization: Orbitals in Harmony
Hybridization, akin to a cosmic choreographer, orchestrates the seamless fusion of atomic orbitals, giving birth to new molecular orbitals that dictate bond lengths, angles, and other intricate molecular features. This dance of hybridized orbitals profoundly influences functional group characteristics.
Molecular Weight: Measuring the Mass of Organic Molecules
In the captivating world of organic chemistry, understanding the molecular weight of organic molecules is akin to deciphering the secret code that governs their behavior. Molecular weight, expressed in atomic mass units (amu), reveals the total mass of all the atoms within a molecule.
Similar to a chef weighing ingredients for a delectable dish, chemists employ molecular weight as a fundamental tool to determine the relative proportions of elements in a molecule. It provides insights into the molecule’s size, shape, and properties, ultimately shaping its role in the symphony of chemical reactions.
The calculation of molecular weight involves summing the atomic masses of all the atoms present in the molecule. Each element’s atomic mass, denoted by its atomic symbol, is readily available from the periodic table. The molecular weight is expressed in amu, where one amu is equivalent to the mass of one atom of carbon-12.
For instance, the molecular weight of methane (CHâ‚„) can be calculated as follows:
Molecular weight of CHâ‚„ = 1(12 amu) + 4(1 amu) = 16 amu
Understanding molecular weight is critical for comprehending the behavior of organic molecules. It serves as a cornerstone for determining their density, volatility, solubility, and reactivity. By unraveling the molecular weight puzzle, chemists gain a deeper appreciation of the intricate dance of atoms within organic molecules.
The Interplay of Molecular Properties in Organic Compounds
In the realm of organic chemistry, understanding the properties of organic molecules is essential for unraveling their behavior and applications. Among these properties, molecular weight, molecular structure, functional groups, shape, size, acidity/basicity, and optical activity are intimately interconnected, forming a complex web of relationships that govern the unique characteristics of each molecule.
Molecular Weight: A Measure of Mass
Molecular weight is a fundamental property that represents the mass of a molecule in atomic mass units (amu). It is directly related to the number and type of atoms present in the molecule’s structure. Larger molecules with more atoms tend to have higher molecular weights.
Molecular Structure: The Foundation of Properties
Molecular structure refers to the arrangement of atoms and their spatial orientation within a molecule. It profoundly influences other properties. For instance, the shape of a molecule is determined by the molecular structure, with symmetrical structures leading to linear, planar, or spherical shapes.
Functional Groups: Building Blocks of Reactivity
Functional groups are specific groups of atoms within a molecule that determine its chemical behavior. They impart characteristic properties depending on their composition and arrangement. Functional groups like alcohols, alkenes, and ketones influence polarity, size, and acidity/basicity.
Polarity: The Dance of Charges
Polarity measures the unequal distribution of electrons within a molecule. It arises from differences in electronegativity between atoms. Polar molecules have a slight positive or negative charge, which affects their interactions with other molecules and their solubility.
Shape and Size: Defining Molecular Geometry
The shape and size of a molecule influence its physical and chemical properties. Larger molecules with complex shapes tend to have lower volatility and solubility compared to smaller, more compact molecules. Shape also plays a crucial role in acidity and basicity.
Acidity/Basicity: Proton Transfer Potential
Acidity and basicity describe a molecule’s ability to donate or accept protons. These properties are influenced by molecular structure, functional groups, and hybridization. Acidic molecules readily release protons, while basic molecules readily accept them.
Optical Activity: Interacting with Light
Optical activity refers to a molecule’s ability to rotate plane-polarized light. It arises from the presence of asymmetric centers or chirality in the molecular structure. Chiral molecules exist as pairs of enantiomers, which are mirror images of each other and exhibit different interactions with chiral environments.
Hybridization: Blending Atomic Orbitals
Hybridization describes the mixing of atomic orbitals to form new hybrid orbitals. The type of hybridization influences the molecular structure, shape, and properties of a molecule. For example, sp³ hybridization results in tetrahedral molecular geometry, while sp² hybridization leads to trigonal planar geometry.
In conclusion, the properties of organic molecules are interconnected and interdependent. By understanding these relationships, we can better predict the behavior and reactivity of these molecules, enabling us to harness their potential for various applications in diverse fields such as medicine, materials science, and energy research.
Polarity: The Dance of Charges in Organic Molecules
Imagine organic molecules as tiny magnets, with positive and negative charges dancing around like partners in a waltz. This dance is what we refer to as polarity. It all starts with a fundamental property called electronegativity, the ability of an atom to attract electrons towards itself. When atoms with different electronegativities come together, they create a tug-of-war, with electrons gravitating more towards the more electronegative atom.
This uneven distribution of electrons creates a separation of charges within the molecule, like two ends of a magnet. The more electronegative atom becomes slightly negative, while the less electronegative atom becomes slightly positive. This separation of charges gives the molecule an overall dipole moment, a measure of its polarity.
The magnitude of the dipole moment depends on the difference in electronegativities and the distance between the charged atoms. The greater the electronegativity difference and the longer the distance between the charges, the more polar the molecule becomes. Highly polar molecules have a strong dipole moment, while nonpolar molecules have a dipole moment close to zero.
Polarity plays a crucial role in many chemical properties of organic molecules, including their solubility, reactivity, and biological activity. Polar molecules have a strong affinity for other polar substances, such as water, while nonpolar molecules prefer to dissolve in nonpolar solvents. Polarity also influences molecular shape and intermolecular forces, affecting the physical and chemical behavior of the molecule. Understanding polarity is therefore essential for comprehending the intricate world of organic molecules.
Explain how molecular structure, functional groups, molecular weight, shape, and solubility are influenced by polarity.
Polarity: The Dance of Charges in Organic Molecules
-
Influence on Molecular Structure:
Polarity creates asymmetrical charge distributions, affecting molecular geometry and bond lengths. Polar functional groups evoke polarity in the entire molecule, influencing its shape and orientation.
-
Impact on Functional Groups:
Functional groups with high polarity (e.g., hydroxyl, carbonyl) create stronger intermolecular forces, influencing reactivity and solubility. Polarity can also amplify the reactivity of functional groups by stabilizing charged intermediates.
-
Relationship with Molecular Weight:
Heavy molecules generally exhibit less polarity due to the increased distribution of charge over a greater volume. However, the presence of highly polar functional groups can counteract this effect.
-
Influence on Shape:
Polarity influences molecular shape by affecting bond angles and bond lengths. Polar bonds tend to straighten out, while nonpolar bonds allow for more flexibility in molecular geometry.
-
Impact on Solubility:
Polar molecules dissolve well in polar solvents due to favorable interactions between the solvent and solute dipoles. On the other hand, nonpolar molecules prefer nonpolar solvents, as there are no significant electrostatic attractions.
Molecular Shape: The Three-Dimensional Architecture of Molecules
In the intricate world of organic chemistry, molecules dance and sway, each with a unique three-dimensional architecture that governs its properties and interactions. Understanding molecular shape is akin to deciphering the secrets of a master sculptor, revealing how the connectivity, bond angles, functional groups, polarity, size, and optical activity intertwine to create a molecule’s distinctive form.
At its core, molecular shape refers to the arrangement of atoms in space. It is not merely an aesthetic consideration but profoundly influences a molecule’s physical and chemical properties. For instance, a compact, spherical molecule like methane tends to be less reactive than an elongated, linear molecule like ethyne. This difference in shape stems from the variation in steric hindrance, which refers to the hindrance posed by bulky atoms or functional groups to the approach of other molecules or reagents.
Bond angles, the angles between adjacent chemical bonds, play a crucial role in determining molecular shape. The tetrahedral geometry of methane, for example, arises from the four bonds formed by the central carbon atom, each directed towards the vertices of a tetrahedron. In contrast, the trigonal planar geometry of carbon dioxide reflects the three bonds formed by the central carbon atom, which lie in a flat plane.
Functional groups, specific groups of atoms that confer characteristic chemical properties on molecules, also impact their shape. The presence of polar functional groups, such as hydroxyl groups (-OH) or amino groups (-NH2), can introduce asymmetry into a molecule, leading to non-linear shapes. These polar groups create regions of high and low electron density, inducing a dipole moment that influences the molecule’s interaction with other molecules and solvents.
Moreover, polarity, the separation of electrical charge within a molecule, is closely tied to molecular shape. Polar molecules, such as water, have a distinct positive end and a negative end. This polarity arises from the uneven distribution of electrons, which can be influenced by the arrangement of atoms and functional groups. The polarity of a molecule affects its solubility, reactivity, and interactions with other polar molecules.
Size, another important factor in molecular shape, refers to the overall volume occupied by a molecule. Larger molecules, with more atoms and functional groups, tend to have more complex shapes and greater steric hindrance compared to smaller molecules. Molecular size influences properties such as diffusion rate, solubility, and the ability to form intermolecular interactions.
Finally, optical activity, the ability of a molecule to rotate plane-polarized light, is another consequence of molecular shape. Optically active molecules are typically chiral, meaning they exist in two non-superimposable mirror-image forms known as enantiomers. The asymmetry in the molecular shape of enantiomers results in their different interactions with polarized light, allowing them to be distinguished and separated.
In summary, molecular shape is a critical concept in understanding the properties and behavior of organic molecules. It provides insights into their reactivity, solubility, interactions, and optical activity. By unraveling the intricate relationships between connectivity, bond angles, functional groups, polarity, size, and optical activity, chemists can gain a deeper appreciation of the molecular world and its complexities.
Molecular Shape: The Three-Dimensional Framework of Organic Molecules
Like an intricate puzzle, the molecular shape of organic compounds arises from the harmonious arrangement of atoms and the bonds that connect them. This three-dimensional architecture is not a mere coincidence; it plays a pivotal role in determining the molecule’s properties and its ability to interact with the surrounding environment.
A Dance of Connectivity and Bond Angles
Imagine a carbon atom gracefully bonding with four other atoms. The angles at which these bonds form dictate the shape of the molecule. For instance, a tetrahedral shape emerges when the bonds are oriented at 109.5 degrees apart. However, if one of the carbon’s bonds is replaced with a double bond, the geometry shifts into a trigonal planar shape. In the case of triple bonds, the molecule adopts a linear configuration.
Functional Groups as Shape-Shifters
Functional groups, like tiny artisans, subtly alter the molecular shape by introducing new atoms and bond arrangements. Alkyl groups, with their hydrocarbon chains, tend to extend the molecule, while carbonyl groups, with their double bonds, introduce bends and kinks. These shape modifications significantly impact the molecule’s overall architecture and influence its reactivity.
Polarity’s Guiding Hand
Polarity, the uneven distribution of electrical charges within a molecule, also plays a role in shaping its form. Polar functional groups, such as hydroxyl (-OH) or carbonyl (-C=O), create regions of positive and negative charges. These charges attract or repel other molecules, influencing the molecule’s orientation and overall shape.
Size and Shape: A Delicate Balance
The size of a molecule is intricately linked to its shape. Smaller molecules, with their compact structures, tend to be more spherical, while larger molecules, with their extended chains or bulky groups, adopt more complex shapes. This interplay between size and shape affects the molecule’s ability to navigate tight spaces or interact with other molecules with specific steric requirements.
Optical Activity: The Dance with Light
For certain molecules, their three-dimensional shape gives rise to a fascinating phenomenon known as optical activity. These molecules, called chiral molecules, exist in two mirror-image forms, like two hands. When light interacts with these molecules, it discriminates between the two forms, resulting in the rotation of polarized light. This optical activity provides valuable insights into the molecular structure and purity of chiral compounds.
Understanding molecular shape is essential for comprehending the behavior and interactions of organic molecules. Its intricate symphony of connectivity, bond angles, functional groups, polarity, size, and optical activity orchestrates the three-dimensional architecture that defines each molecule’s unique identity.
Size: The Expansive Nature of Organic Molecules
As we delve deeper into the captivating world of organic molecules, we encounter the intriguing concept of molecular size. This fundamental property refers to the physical dimensions of a molecule, its sheer expanse in the molecular realm.
A Deeper Dive into Molecular Size
Molecular size is intricately intertwined with molecular structure, the arrangement of atoms and bonds within a molecule. Larger molecules boast a greater number of constituent atoms, forming intricate scaffolds of atomic connections. Smaller molecules, on the other hand, are more compact and possess a reduced atomic count.
The Influence of Functional Groups
Functional groups, the distinctive molecular fragments that determine chemical reactivity and properties, also play a pivotal role in defining molecular size. Bulky functional groups, such as aromatic rings or branched alkyl chains, contribute to increased molecular dimensions. Conversely, smaller functional groups, such as simple alkyl groups or halogens, tend to occupy less space within a molecule.
Shape Matters
Molecular shape is another factor that influences molecular size. Linear molecules, with their atoms arranged in a straight line, are inherently more elongated than branched molecules, which exhibit a more compact and voluminous structure.
Size and Solubility: A Delicate Balance
Molecular size exerts a significant impact on solubility, the ability of a substance to dissolve in a solvent. Generally, smaller molecules exhibit greater solubility as they experience less steric hindrance, which refers to the hindrance caused by the spatial arrangement of atoms within a molecule.
The Impact of Molecular Size on Organic Molecules
Size matters, even in the realm of organic molecules. The molecular size of a molecule, often measured in atomic mass units (amu), profoundly influences its functional groups, molecular weight, shape, and solubility.
Functional Groups
Functional groups are the atoms and atom groups that impart characteristic chemical properties to organic molecules, such as hydroxyl (-OH) for alcohols and amine (-NH2) for amines. Larger molecules typically accommodate a greater number of functional groups, allowing for a broader range of reactions and chemical diversity.
Molecular Weight
In general, larger molecules possess higher molecular weights, as they contain more atoms. This increased molecular weight can alter the physical properties of the molecule, including melting point, boiling point, and density.
Shape
The shape of an organic molecule determines its geometry and can also influence its reactivity. Larger molecules tend to adopt more complex shapes, such as branched chains or cyclic structures, which can lead to different steric effects and alter the molecule’s interactions with other molecules.
Solubility
Solubility refers to the ability of a substance to dissolve in a solvent. Smaller molecules are generally more soluble in most solvents because they can more easily interact with and disperse among the solvent molecules. Larger molecules, on the other hand, may have difficulty dissolving due to their increased size and decreased surface area.
Define solubility and discuss its importance in organic chemistry.
Solubility: The Key to Understanding Organic Molecules
In the tapestry of organic chemistry, solubility plays a pivotal role in unraveling the intricate relationships between molecules and their interactions with the world around them. It is the ability of a substance to dissolve in a solvent, forming a homogeneous mixture. This fascinating phenomenon dictates how organic molecules behave in various chemical reactions, biological processes, and everyday applications.
Solubility is a manifestation of the delicate balance between molecular structure and solvent properties. Polar molecules, with their uneven distribution of charge, tend to dissolve in polar solvents like water. Nonpolar molecules, on the other hand, prefer nonpolar solvents such as benzene or hexane. This principle stems from the fundamental concept of “like dissolves like.”
The importance of solubility in organic chemistry cannot be overstated. It allows chemists to:
- Separate and purify organic compounds: By leveraging differences in solubility, scientists can selectively extract target molecules from complex mixtures.
- Control chemical reactions: Solubility influences the rates and equilibria of chemical reactions by affecting the availability of reactants and the formation of products.
- Design drugs and therapeutic agents: Solubility plays a crucial role in determining the bioavailability, distribution, and efficacy of drugs within the body.
Delving into the molecular factors that influence solubility, we encounter a symphony of interactions. Functional groups, the reactive centers of organic molecules, exert a profound effect on their solubility. For instance, hydroxyl (-OH) groups increase polarity and thus enhance solubility in water. Molecular weight also plays a role, as larger molecules tend to be less soluble than their smaller counterparts.
Beyond these structural considerations, the shape and size of organic molecules also contribute to solubility. Compact molecules generally dissolve more readily than those with elongated or bulky structures. Polarity and hybridization further modulate solubility by influencing the molecule’s ability to interact with solvent molecules.
Understanding the principles of solubility is essential for navigating the intricate world of organic chemistry. By unraveling these relationships, chemists gain the power to predict, control, and manipulate the behavior of organic molecules in a wide range of applications, from designing new materials to developing life-saving pharmaceuticals.
Solubility: The Compatibility of Organic Molecules in Solvents
Within the intricate realm of organic chemistry, solubility emerges as a captivating concept that unveils the compatibility of organic molecules with various solvents. This exceptional property governs the ability of organic compounds to dissolve and form homogeneous mixtures, revealing their molecular characteristics and influencing numerous chemical processes.
Molecular Structure
The molecular structure of an organic molecule plays a pivotal role in determining its solubility. Branched and bulky structures, characterized by increased surface area, tend to be less soluble than their compact, linear counterparts. This reduced solubility stems from the hindered intermolecular interactions that arise from the increased steric hindrance.
Functional Groups
Functional groups exert a significant influence on solubility. Polar functional groups, such as hydroxyl (-OH) and amino (-NH2) groups, establish strong hydrogen bonds with water molecules, enhancing their solubility in aqueous solvents. In contrast, nonpolar functional groups, such as hydrocarbons (-CH3), lack the ability to form hydrogen bonds and exhibit limited solubility in polar solvents.
Molecular Weight
Molecular weight also contributes to solubility. Smaller, lighter molecules possess greater kinetic energy, allowing them to overcome intermolecular forces more efficiently. Consequently, they tend to be more soluble than larger, heavier molecules.
Polarity
Polarity influences solubility through intermolecular interactions. Polar molecules, characterized by uneven charge distribution, interact favorably with polar solvents, forming dipole-dipole interactions. On the other hand, nonpolar molecules interact more effectively with nonpolar solvents, due to van der Waals forces.
Shape
The shape of an organic molecule can impact solubility. Molecules with compact, spherical shapes exhibit greater surface area, facilitating interactions with solvent molecules and enhancing solubility. Elongated, rod-like molecules, on the other hand, experience reduced solubility due to their diminished surface area.
Size
Size is an important factor in solubility. Smaller molecules, with their ability to penetrate solvent molecules more easily, tend to be more soluble than larger molecules. This reduced steric hindrance allows for more efficient intermolecular interactions.
Unveiling the intricate relationship between these molecular characteristics and solubility empowers chemists to predict the behavior of organic compounds in diverse solvents. This understanding underpins numerous applications in fields such as pharmaceutical development, where solubility is crucial for drug delivery and absorption.
Understanding the Proton-Transfer Dance: Acidity and Basicity in Organic Molecules
Acidity and basicity are essential concepts for grasping the chemical behavior of organic molecules. These properties revolve around the transfer of protons, the positively charged hydrogen ions. Comprehending the driving forces behind acidity and basicity is crucial for unraveling the fascinating world of organic chemistry.
In essence, acidity measures a molecule’s tendency to donate a proton, while basicity indicates its ability to accept a proton. This protonic dance is driven by the stability of the resulting ions. When a molecule gives up a proton, it forms a negative species known as an anion, while the proton’s recipient becomes a positively charged cation. The stability of these ions dictates the acidity and basicity of the organic molecule.
Factors influencing acidity and basicity include the molecule’s molecular structure, functional groups, polarity, hybridization, and **optical activity. For instance, molecules with electronegative atoms (such as oxygen or nitrogen) tend to withdraw electron density, making the attached hydrogen more acidic. Conversely, molecules with electron-donating groups (such as alkyl groups) reduce the acidity of the hydrogen atoms.
Understanding acidity and basicity is not merely an academic pursuit. It underpins the chemical reactions that drive life processes, from enzyme catalysis to acid-base reactions in the digestive system. By delving into the world of proton transfer, we unlock the secrets that govern the behavior of organic molecules, enabling us to harness their potential for medicine, materials science, and beyond.
How Molecular Structure, Functional Groups, Polarity, Hybridization, and Optical Activity Influence Protonation and Deprotonation
Chemical reactions involving the transfer of protons (H+ ions) play a crucial role in organic chemistry. The ability of organic molecules to undergo protonation (gain a proton) or deprotonation (lose a proton) is governed by a complex interplay of molecular properties. Let’s delve into how these factors influence protonation and deprotonation.
Molecular Structure
The molecular structure of an organic molecule influences its acidity and basicity. Molecules with more electronegative atoms (like oxygen, nitrogen, or halogens) have higher electron-withdrawing properties, making them more likely to withdraw electrons from hydrogen atoms and form acidic protons.
Functional Groups
Functional groups are specific atomic arrangements within a molecule that confer characteristic chemical properties. For example, carboxylic acid functional groups (-COOH) contain highly acidic protons due to the presence of an electronegative oxygen atom. Conversely, amine functional groups (-NH2) contain basic nitrogen atoms that can accept protons.
Polarity
The polarity of a molecule refers to the uneven distribution of electrons. Molecules with polar functional groups exhibit a localized charge separation, creating a dipole moment. This polarity influences the strength of protonation and deprotonation. For instance, acidic protons are more likely to be donated to highly electronegative atoms, while basic protons are more likely to be accepted by electronegative atoms.
Hybridization
The hybridization of atoms in a molecule affects the shape of the molecular orbitals. Molecules with sp3 hybridized carbon atoms have tetrahedral geometry, while molecules with sp2 hybridized carbon atoms have trigonal planar geometry. This hybridization also influences the acidity and basicity of protons. For example, sp2 hybridized carbon atoms are more acidic than sp3 hybridized carbon atoms due to the greater s-character of the sp2 orbitals.
Optical Activity
Optical activity is the ability of a molecule to rotate plane-polarized light. Molecules that exhibit optical activity are chiral, meaning they are not superimposable on their mirror images. Chiral molecules can contain asymmetric carbon atoms or other structural features that create non-superimposable mirror images. Optical activity influences protonation and deprotonation kinetics, as the orientation of the chiral center can affect the accessibility of the acidic or basic proton to the proton donor or acceptor.
Define optical activity and explain its origins in molecular asymmetry.
Optical Activity: The Dance of Molecules with Light
In the captivating realm of organic chemistry, where molecules tell their stories through their structures, one fascinating aspect that unfolds is their ability to interact with light in an enchanting dance known as optical activity. This extraordinary phenomenon has captivated scientists and chemists alike, revealing the intricate relationship between the molecular world and the ethereal realm of light.
At the heart of optical activity lies a concept called molecular asymmetry. Imagine a molecule as a three-dimensional sculpture, with its atoms arranged in a chiral configuration. Chiral molecules, like our two hands, are non-superimposable mirror images of each other. It’s this asymmetrical architecture that enables them to dance with light in a mesmerizing way.
When chiral molecules encounter polarized light, which consists of electromagnetic waves vibrating in a specific plane, they interact in a selective manner. One enantiomer (mirror image) of the molecule rotates the plane of polarized light clockwise, while its counterpart rotates it counterclockwise. This remarkable ability allows us to differentiate between molecules that are otherwise identical in their chemical composition.
From medicinal chemistry to materials science, optical activity holds immense importance. By harnessing the unique properties of chiral molecules, scientists can design drugs that selectively target specific biological receptors, leading to improved efficacy and reduced side effects. In materials science, optically active molecules find application in the development of advanced optical materials, such as liquid crystals and chiral catalysts.
Understanding the intricate relationship between molecular structure and optical activity provides a powerful tool for unraveling the secrets of the molecular world. As we delve deeper into this fascinating domain, we uncover the profound influence that molecular asymmetry has on the interactions that shape our universe.
Discuss how molecular structure, functional groups, polarity, shape, and size are related to optical activity.
Optical Activity: The Dance of Light and Molecules
Among the intriguing characteristics of organic molecules lies their ability to interact with light in a fascinating way known as optical activity. This phenomenon arises when a molecule possesses an asymmetrical structure, meaning it cannot be superimposed on its mirror image. Such molecules are said to be chiral and exhibit the ability to polarize light, rotating its plane of polarization either clockwise or counterclockwise.
This optical activity is profoundly influenced by the molecule’s molecular structure. For instance, molecules containing stereogenic centers, or asymmetric carbon atoms bonded to four different groups, often display optical activity. The arrangement of functional groups around these stereogenic centers contributes to the overall molecular shape and symmetry, thereby dictating the molecule’s interaction with light.
Functional groups, with their diverse electronic properties, also play a pivotal role in optical activity. Conjugated double bonds and aromatic rings can influence the extent and direction of light rotation. These functional groups can interact with light through electronic transitions, causing changes in the molecule’s polarizability and, consequently, its optical activity.
Moreover, polarity within the molecule affects its interaction with light. Polar groups can create localized electric fields that influence the polarization of light. The orientation of these polar groups relative to the light’s path determines the direction of rotation.
Finally, molecular size and shape can also affect optical activity. Larger molecules with complex structures tend to exhibit more pronounced optical activity than smaller, simpler molecules. Additionally, molecular shape can influence the orientation of functional groups and determine the extent to which they interact with light.
Hybridization: The Alchemists of Molecular Orbitals
In the realm of organic chemistry, hybridization plays the role of a master alchemist, transforming atomic orbitals into molecular ones. Imagine atomic orbitals as individual building blocks, each possessing a unique shape and energy level. Hybridization is the magical process that fuses these building blocks, creating hybrid orbitals that possess entirely new properties.
This mystical union between atomic orbitals occurs when atoms strive to achieve the most stable electronic configuration. The electrons, eager to arrange themselves in a manner that minimizes their energy, dance around the atomic nuclei like celestial bodies in an intricate cosmic ballet. Hybridization orchestrates this celestial harmony, aligning the atomic orbitals in a way that optimizes electron stability.
The result of this orbital metamorphosis is the birth of hybrid orbitals, which defy their atomic origins and assume new shapes and orientations. These hybrid orbitals, like shapeshifting Transformers, mold themselves into specific geometries, paving the way for the formation of molecular bonds. The type of hybridization an atom undergoes depends on the number and type of atomic orbitals it possesses, as well as the number and type of bonds it forms.
Hybridization is the architect of molecular structure, influencing a molecule’s geometry, polarity, and reactivity. It governs the angles between bonds, the spatial arrangement of atoms, and the overall shape of the molecule. Hybridization is the unseen hand that sculpts the molecules we encounter in the world around us.
How Hybridization Shapes the World of Organic Molecules
Imagine a vibrant world where tiny organic molecules dance and interact, each one carrying a unique identity shaped by its molecular structure. Within this realm, hybridization emerges as the master architect, orchestrating the arrangement of atoms and dictating the molecule’s destiny.
The Birth of Hybrid Orbitals
Hybridization begins when atoms don’t play by the rules. Rather than using their standard atomic orbitals, they borrow a trick from quantum mechanics and blend them together, creating a new breed of orbitals known as hybrid orbitals. These hybrid orbitals have a distinct shape and energy level, reflecting the number and type of atoms involved in the hybridization.
Hybridization’s Impact on Molecular Structure
The choice of hybridization has a profound effect on the molecular structure. For instance, in methane (CH4), carbon’s four valence electrons form four sp³ hybrid orbitals, resulting in a tetrahedral shape. In contrast, in ethylene (C2H4), carbon’s two valence electrons form three sp² hybrid orbitals, leading to a trigonal planar geometry.
Functional Groups: The Building Blocks of Chemistry
Functional groups serve as the language of organic chemistry, determining a molecule’s chemical behavior. Hybridization influences the bonding within functional groups, affecting their polarity and reactivity. For example, in alcohols (R-OH), the hybridization of the oxygen atom affects the polarity of the O-H bond, making alcohols acidic.
Polarity: The Balancing Act
Polarity reflects the uneven distribution of electrons within a molecule. Hybridization affects polarity by shaping the electronegativity of atoms. For instance, in water (H2O), the sp³ hybridization of oxygen creates a more electronegative oxygen atom, leading to a polar molecule.
Acidity/Basicity: The Duel of Proton Transfer
Acidity and basicity describe a molecule’s ability to donate or accept protons. Hybridization plays a crucial role by altering the availability of electrons for protonation or deprotonation. For example, in carboxylic acids (R-COOH), the sp³ hybridization of the carbon atom facilitates the loss of a proton, making them acidic.
Hybridization stands as a cornerstone of organic chemistry, shaping the molecular structure, functional groups, polarity, acidity, and basicity of molecules. By understanding the nuances of hybridization, we unlock the secrets of organic molecules, deciphering their chemical behaviors and paving the way for advancements in fields from medicine to materials science.