Unveiling The Orchestral Regulation Of Gene Expression In Eukaryotes: A Comprehensive Guide
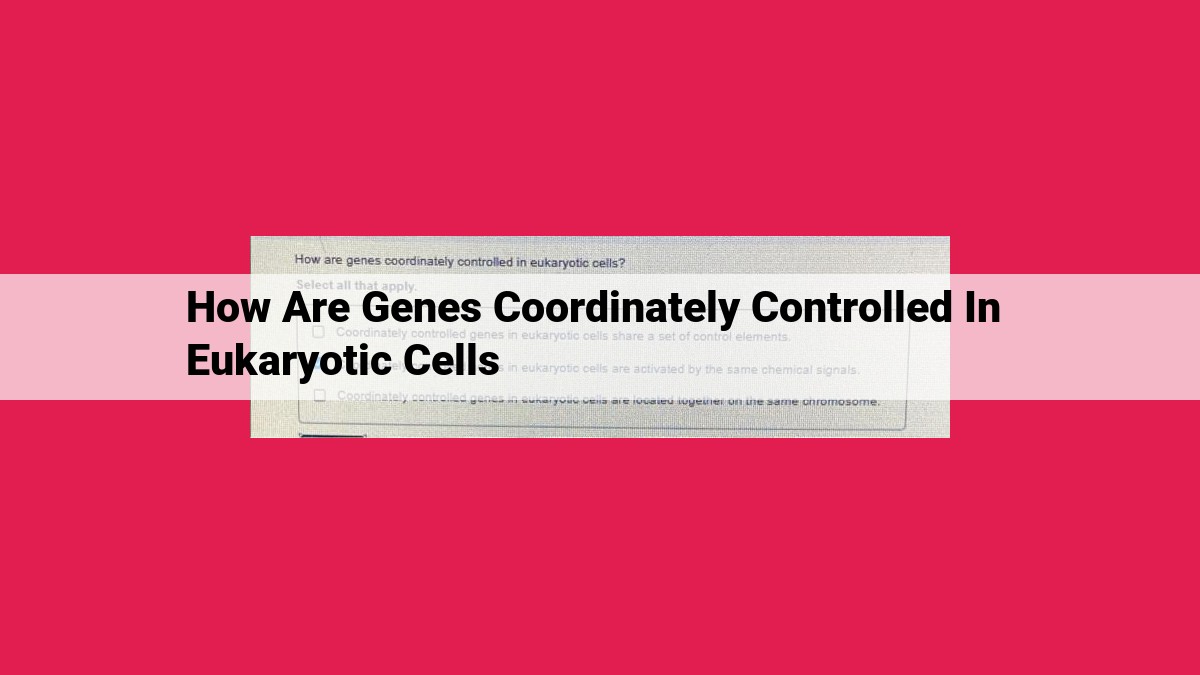
Eukaryotic gene coordination involves a symphony of regulatory mechanisms: enhancers, promoters, silencers, and insulators control transcription; histone modifications and DNA methylation shape chromatin accessibility; transcription factors bind regulatory elements and recruit coactivators or corepressors; gene looping brings distant elements into proximity; and non-coding RNAs influence gene expression. This intricate interplay ensures the proper functioning and development of eukaryotic cells.
Regulatory Elements: Guardians of Gene Expression
In the intricate world of gene regulation, regulatory elements serve as gatekeepers, orchestrating the precise expression of genetic information. These specialized DNA sequences, strategically positioned along the genome, play a crucial role in determining which genes are activated and when.
Among these guardians, enhancers stand out as powerful activators, binding to transcription factors and boosting gene activity from afar. Promoters, located immediately upstream of genes, provide the crucial docking site for RNA polymerase, the enzyme that transcribes DNA into RNA. Conversely, silencers act as repressors, binding to proteins that prevent gene transcription.
An additional layer of control is exerted by insulators, which function as barriers, blocking the influence of regulatory elements on nearby genes. By preventing the spread of transcriptional signals, insulators maintain the integrity of gene expression domains, ensuring that each gene is regulated independently.
Together, these regulatory elements form a complex interplay, meticulously controlling the timing and level of gene transcription. Their precise coordination ensures that cells can respond appropriately to environmental cues, differentiate into specialized cell types, and maintain proper physiological function.
Chromatin Modifiers: Shaping the Accessibility Landscape
In the molecular orchestra that regulates gene expression, chromatin modifiers play a pivotal role in shaping the accessibility landscape of DNA. These maestros wield their power through intricate mechanisms involving histone modifications and DNA methylation, influencing the structure and function of chromatin, the tightly packed complex of DNA and proteins that houses our genetic information.
Histone Modifications: The Dance of Chemical Tags
Histones, the protein building blocks of chromatin, are adorned with an array of chemical tags, like musical notes, that influence the accessibility of DNA. These modifications, such as acetylation, methylation, and phosphorylation, alter the electrostatic charge and structural dynamics of histones, affecting how tightly they bind to DNA.
Acetylated histones, for instance, resemble jubilant dancers, attracting proteins that promote gene expression. They loosen the chromatin structure, making DNA more accessible to the molecular players responsible for transcription, the process of converting DNA into RNA. Conversely, methylated histones act like stern chaperones, repressing gene expression by condensing chromatin and inhibiting access to DNA.
DNA Methylation: A Symphony of Silencing
DNA methylation, a more permanent modification, adds a chemical mark to the DNA itself, primarily at CpG islands. These methyl groups act as roadblocks, preventing the binding of transcription factors and silencing gene expression. DNA methylation is crucial for embryonic development, X-chromosome inactivation, and the suppression of transposable elements, the remnants of ancient viruses that can disrupt gene function.
Chromatin Modifiers: Masters of Accessibility
Chromatin modifiers, such as histone acetyltransferases (HATs) and histone deacetylases (HDACs), switch between these chemical tags, modulating chromatin structure and gene expression accordingly. DNA methyltransferases (DNMTs) and TET proteins control DNA methylation, ensuring the proper silencing and activation of genes.
Chromatin remodeling complexes, like SWI/SNF and NuRD, use ATP hydrolysis as fuel to alter chromatin structure, either opening up or compacting DNA to regulate gene access. These complexes work in concert with other regulatory factors, such as transcription factors, to fine-tune gene expression, ensuring the harmonious coordination of cellular functions.
Implications in Health and Disease
Understanding chromatin modifiers and their role in gene regulation has profound implications for health and disease. Aberrant chromatin modifications have been linked to cancer, developmental disorders, and neurological diseases. For instance, mutations in HATs and HDACs can lead to gene dysregulation, contributing to tumorigenesis and mental health disorders.
Targeting chromatin modifiers with drugs is an emerging therapeutic strategy for a wide range of diseases. By manipulating chromatin structure and gene expression, these drugs aim to restore cellular balance and alleviate disease symptoms. As research continues, chromatin modifiers are set to play an increasingly central role in advancing personalized medicine and improving human health.
Transcription Factors: The Key Orchestrators of Gene Activation
In the orchestra of gene expression, transcription factors are the masterful conductors, directing the symphony of cellular processes. These specialized proteins act as messengers, bridging the gap between regulatory DNA elements and the machinery that transcribes genes into RNA. They possess a unique ability to bind to specific DNA sequences called regulatory elements, which flank genes and control their activity.
Upon binding to these regulatory elements, transcription factors recruit a cast of supporting proteins, including coactivators and corepressors. These partners form a complex that either enhances or represses gene expression, depending on their composition. Coactivators, like skilled musicians, amplify the transcription factor’s signal, promoting gene activation. Corepressors, on the other hand, act as silencers, dampening the transcription factor’s message and inhibiting gene expression.
The interplay between these proteins determines the level of gene activity. For example, a transcription factor may bind to a promoter element, but if it recruits more corepressors than coactivators, the gene will remain silenced. Conversely, a high ratio of coactivators to corepressors will result in robust gene activation.
Transcription factors orchestrate gene expression in response to a myriad of signals. They can be activated by environmental cues, such as hormones or growth factors, or by internal cellular events. By controlling the expression of specific genes, transcription factors fine-tune cellular responses, ensuring that cells function appropriately in different contexts.
Their versatility extends beyond isolated gene regulation. Transcription factors also engage in complex interactions with each other, forming intricate regulatory networks. These networks allow for precise control of gene expression programs, ensuring that genes are activated or repressed in a coordinated fashion, like a harmonious symphony.
In essence, transcription factors are the maestros of gene expression, wielding their power to conduct the cellular orchestra. Their meticulous coordination and interactions ensure that the genetic symphony plays out smoothly, giving rise to the intricate processes that govern life.
Coactivators and Corepressors: The Supporting Cast
In the gene expression orchestra, transcription factors take center stage, directing the flow of genetic information. But behind these stars, an essential supporting cast of coactivators and corepressors plays a crucial role in fine-tuning gene expression.
Coactivators: The Enhancers
Coactivators are like the eager assistants of transcription factors. They bind to transcription factors, providing a boost of energy to drive gene activation. These molecular helpers form a molecular bridge between transcription factors and the RNA polymerase machinery, facilitating the initiation of transcription. They act as a catalyst, enhancing the ability of transcription factors to turn genes on.
Corepressors: The Silencers
On the other side of the stage, corepressors serve as the silencers of gene expression. They are the antagonists of coactivators, binding to transcription factors to block their action and inhibit gene activation. Corepressors form a molecular barrier between transcription factors and the RNA polymerase machinery, preventing the initiation of transcription. They act as a brake, repressing the expression of genes that are not needed at that particular moment.
The Interplay: A Delicate Balance
Coactivators and corepressors engage in a constant tug-of-war, ensuring a delicate balance in gene expression. The ratio of coactivators to corepressors determines the level of gene activation or repression. This interplay is essential for maintaining cellular homeostasis and responding to changing environmental cues.
In summary, coactivators and corepressors are the unsung heroes of gene regulation. They work in concert with transcription factors to fine-tune gene expression, ensuring the precise coordination of cellular processes and the proper functioning of eukaryotic cells.
Gene Looping: The Dance that Brings Genes Together
In the intricate symphony of our cells, genes, like musical notes, hold melodies of life. However, these melodies are not always played in isolation. Sometimes, notes must dance together, intertwining their tunes to produce a harmonious sound. This is where gene looping steps in, the graceful ballet that brings distant regulatory elements into close proximity with promoters, orchestrating a flawless performance of gene expression.
Gene looping, a molecular ballet, is performed by a carefully orchestrated troupe of proteins. These proteins bend and mold the DNA, creating graceful loops that connect distant regulatory elements, such as enhancers and silencers, to the DNA’s指揮棒, the promoters. These regulatory elements are like master switches, determining whether a particular gene is turned on or off.
Once the loop is formed, the regulatory elements can directly interact with the promoters, whispering secrets that turn on or silence the gene. This enchanting dance allows for precise control of gene expression, ensuring that only the right genes are activated at the right time, in the right place.
Gene looping is not just a graceful dance; it’s a vital component of our genetic repertoire. It allows cells to respond rapidly to changing environments, fine-tuning gene expression to meet the demands of every moment. This molecular ballet is a testament to the exquisite choreography of life, where each step brings us closer to understanding the complexities of our genetic symphony.
Non-coding RNAs: The Hidden Powerhouses of Gene Regulation
Gene expression is a complex and tightly controlled process essential for the proper functioning and development of eukaryotic cells. While DNA sequences encode the instructions for proteins, non-coding RNAs (ncRNAs) play a crucial role in regulating gene expression, acting as hidden players in the intricate symphony of genetic control.
There are two main types of ncRNAs involved in gene regulation: microRNAs (miRNAs) and long non-coding RNAs (lncRNAs). MiRNAs, tiny RNA molecules of about 20-25 nucleotides, are known for their ability to silence gene expression by binding to specific sequences in messenger RNA (mRNA), effectively preventing its translation into protein.
LncRNAs, on the other hand, are much longer, ranging from 200 to thousands of nucleotides. They exert their regulatory influence through various mechanisms, including:
-
Interaction with transcription factors: LncRNAs can bind to transcription factors, influencing their activity and recruitment to specific genes. For example, the lncRNA HOTAIR can bind to the transcription factor PRC2, directing it to repress gene expression in certain regions of the genome.
-
Influence on gene looping: LncRNAs can bridge distant regulatory elements and promoters, bringing them into close proximity and facilitating gene expression. The lncRNA H19 is known to loop the beta-globin gene cluster, promoting its transcription.
ncRNAs also interact with each other, forming complex regulatory networks that fine-tune gene expression. For instance, miRNAs can target and degrade lncRNAs, modulating their regulatory effects.
The discovery of ncRNAs and their role in gene regulation has revolutionized our understanding of how genes are controlled. These hidden players orchestrate a sophisticated regulatory system, ensuring the precise and coordinated expression of genes that is essential for cellular harmony.