Optimizing Blood Flow: Understanding The Pressure Gradient, Resistance, And Viscosity
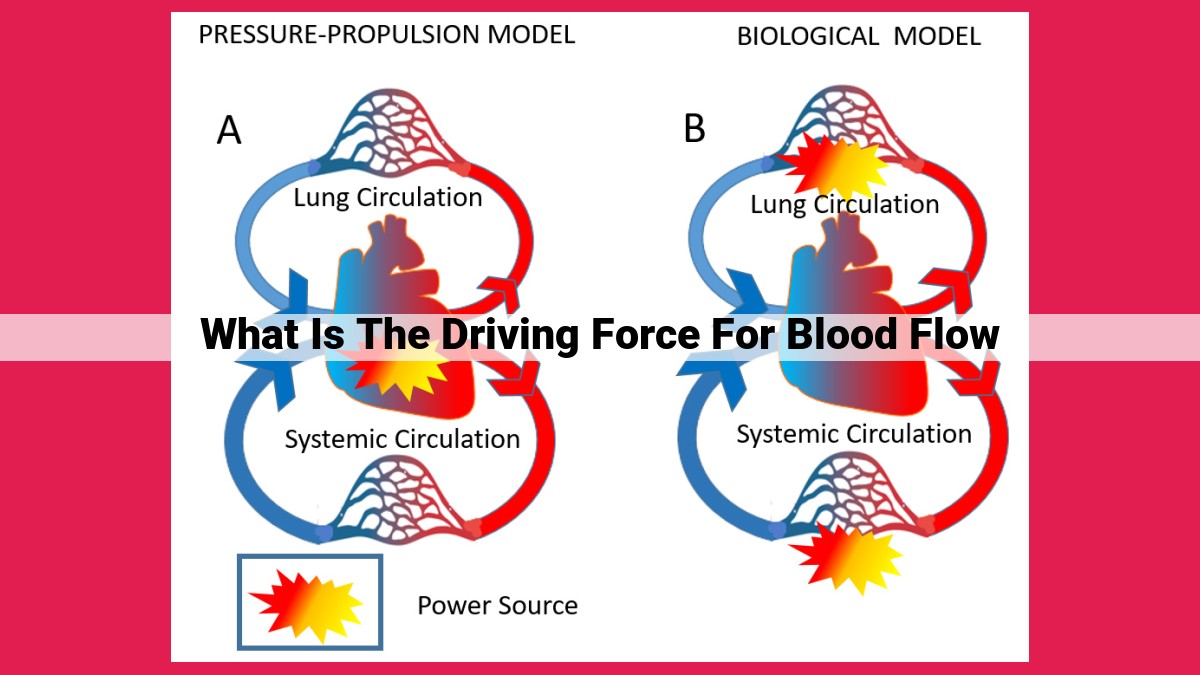
Blood flow is driven by a pressure gradient, the difference in pressure between two points. This gradient forces blood to move from areas of high pressure to low pressure. Resistance, such as the friction of blood against blood vessel walls, impedes blood flow. Viscosity, the thickness of blood, also affects flow rate. Poiseuille’s Law quantifies the relationship between pressure gradient, resistance, and flow rate. Blood flow is crucial for cardiovascular health, as it transports oxygen, nutrients, and hormones throughout the body.
Pressure Gradient: The Driving Force
- Define pressure gradient and explain its role in blood flow.
- Discuss the concept of hydrostatic pressure and hydraulic head.
Pressure Gradient: The Driving Force Behind Blood Flow
Imagine a river’s steady flow, driven by the relentless pull of gravity. In our bodies, a similar force guides the tireless journey of blood through our vessels: the pressure gradient.
Pressure Gradient: The Guiding Force
Picture a pipe filled with fluid. The pressure at one end is higher than at the other, creating a difference or gradient. This pressure gradient drives the fluid forward, just as gravity compels water to flow downhill.
In our cardiovascular system, the pressure gradient refers to the difference in pressure between two points in a blood vessel. It acts as the primary force that propels blood through the intricate network of arteries, capillaries, and veins.
Hydrostatic Pressure and Hydraulic Head
Hydrostatic pressure is the pressure exerted by a fluid due to its weight. Hydraulic head is the vertical distance between two points in a fluid. In the context of blood flow, the hydraulic head is the difference in elevation between two points in the circulatory system.
The interplay between hydrostatic pressure and hydraulic head significantly influences the pressure gradient. For instance, a greater hydraulic head (such as when standing upright) increases the hydrostatic pressure and, consequently, the pressure gradient.
Understanding the pressure gradient is crucial for grasping the dynamics of blood flow in our bodies. It governs the rate and direction of circulation, ensuring that oxygen and nutrients reach every corner of our being.
Resistance: The Obstacle Course for Blood Flow
As blood embarks on its journey through the body’s intricate vascular network, it encounters an ever-present challenge: resistance, the friction that hampers its smooth passage. Resistance poses a formidable obstacle, influencing blood flow dynamics and playing a crucial role in maintaining cardiovascular health.
Factors Shaping Resistance
Resistance stems from the interaction of blood with the blood vessel walls. Several factors contribute to its magnitude:
- Vessel Length: Longer blood vessels present a more extended obstacle course for blood, increasing resistance.
- Vessel Diameter: Narrower vessels exert greater resistance due to the increased friction between blood and the vessel wall.
- Blood Viscosity: Thicker blood, with higher viscosity, flows with greater difficulty, contributing to higher resistance.
Related Concepts: Impedance, Friction, Obstruction
Resistance is closely intertwined with other concepts that govern blood flow:
- Impedance: A broader term encompassing all factors that impede blood flow, including resistance, inertia, and capacitance.
- Friction: The force opposing the passage of blood through the blood vessel walls.
- Obstruction: A complete blockage of blood flow, often caused by clots or plaque buildup.
Understanding these related concepts provides a more comprehensive picture of the challenges faced by blood as it navigates the body’s circulatory system.
Viscosity: Thickness Matters
Imagine blood as a river flowing through the intricate network of our cardiovascular system. Like any river, blood’s flow is influenced by various factors, one of which is viscosity.
What is Viscosity?
Viscosity, in essence, is the thickness of a fluid. It measures the resistance to flow, akin to how thick oil flows more slowly than water. Blood, a complex fluid, has a higher viscosity than water, which affects its flow dynamics.
Impact on Blood Flow
Viscosity plays a crucial role in blood flow. Thicker blood, with high viscosity, flows more slowly. This can lead to increased pressure in the blood vessels, making the heart work harder to pump blood. In contrast, thinner blood flows more easily, reducing the strain on the heart.
Shear Stress and Rate
Viscosity is closely related to shear stress and shear rate. Shear stress is the force applied to a fluid when it flows, while shear rate is the rate at which fluid layers move past each other. In blood flow, shear stress is higher when viscosity is high.
Newtonian Fluids
Blood is considered a Newtonian fluid, meaning its viscosity remains constant at varying shear rates. This is in contrast to non-Newtonian fluids, whose viscosity changes with shear rate.
Implications for Cardiovascular Health
Understanding viscosity is essential for cardiovascular health. High blood viscosity can increase the risk of heart disease, stroke, and blood clots. Factors such as high cholesterol, dehydration, and low red blood cell count can all increase blood viscosity.
Maintaining Healthy Viscosity
Maintaining healthy blood viscosity is crucial for optimal heart health. Staying hydrated, exercising regularly, and eating a balanced diet can help keep blood viscosity in check. Additionally, certain medications can be used to lower blood viscosity if必要.
Viscosity is a crucial factor influencing blood flow. Understanding its impact on blood flow is essential for maintaining cardiovascular health. By addressing factors that can affect viscosity, we can help ensure that the blood flow through our bodies remains smooth and efficient.
**Understanding Flow Rate: Measuring the River of Blood**
In the intricate world of human physiology, blood flows like a mighty river, delivering life-sustaining oxygen and nutrients to every corner of our bodies. Flow rate is a crucial parameter that measures the pace of this vital movement.
Simply put, flow rate refers to the volume of blood passing through a given point over a specific time interval. It is a measure of how much blood is transported, and is expressed in units such as milliliters per minute (mL/min).
Flow rate is of utmost significance because it influences the delivery of essential substances to our tissues. Insufficient flow rate can lead to tissue ischemia, while excessive flow rate can damage blood vessels.
There are three main types of flow rate:
1. Volume Flow Rate: Measures the volume of blood flowing per unit time. This is the most commonly used type in medical applications.
2. Mass Flow Rate: Measures the mass of blood flowing per unit time. It is often used in research settings to determine the total amount of oxygen delivered.
3. Volumetric Flow Rate: Measures the volume of blood transported per unit time across a specific cross-sectional area. This is frequently used in hemodynamics to analyze blood flow patterns.
Understanding flow rate is essential for cardiovascular health. Abnormal flow rates can be indicative of underlying medical conditions, such as heart disease, atherosclerosis, or anemia. By measuring flow rate, doctors can assess the health of the circulatory system and make informed decisions about appropriate treatment options.
Poiseuille’s Law: The Formula for Blood Flow
Understanding the intricacies of blood flow is crucial for maintaining cardiovascular health. Poiseuille’s Law is a fundamental principle that governs the flow of blood in vessels. It provides a quantitative description of how various factors influence the rate at which blood flows through the body.
Poiseuille’s Law states that the volume flow rate (Q) of a fluid through a cylindrical vessel is directly proportional to the pressure gradient (ΔP) driving the flow, the fourth power of the vessel’s radius (r), and inversely proportional to the fluid’s viscosity (η). Mathematically, it can be expressed as:
Q = (ΔP * πr^4) / (8ηL)
where L represents the length of the vessel.
The Hagen-Poiseuille equation, a more detailed form of Poiseuille’s Law, incorporates additional fluid dynamic concepts. It accounts for the shear stress acting on the fluid and the parabolic velocity profile within the vessel.
Laminar flow, a type of fluid flow in which layers of fluid move smoothly past each other, is assumed in Poiseuille’s Law. This type of flow is characteristic of blood flow in small vessels. As blood velocity increases or vessel size decreases, the flow may transition to turbulent flow, where layers of fluid become highly disrupted and unpredictable.
Understanding the implications of Poiseuille’s Law is essential for comprehending cardiovascular physiology. It helps explain why vasodilation (widening of blood vessels) increases blood flow, while vasoconstriction (narrowing of blood vessels) decreases it. Additionally, it demonstrates the importance of maintaining proper blood viscosity to ensure adequate flow and oxygen delivery to tissues.
The Dynamic Balance: Driving Force and Resistance
In the intricate network of our circulatory system, a delicate dance unfolds between opposing forces that govern the flow of blood. On one end, a pressure gradient acts as the driving force, propelling blood along the vessels. On the other, resistance serves as an obstacle course, slowing down the flow.
The pressure gradient, a difference in pressure between two points, is essential for blood movement. It creates a push that drives blood from areas of higher pressure to areas of lower pressure. The greater the pressure difference, the stronger the driving force and the faster the flow.
However, the journey of blood through the body is not without challenges. As blood navigates the intricate web of arteries, capillaries, and veins, it encounters resistance. Resistance stems from various factors, including the narrowness of the vessels, the presence of obstacles such as plaque, and the viscosity of the blood itself.
Just as a runner faces resistance from terrain and obstacles, blood flow encounters resistance from the friction against vessel walls and the turbulence created by flow disruptions. This resistance impairs the flow rate, reducing the volume of blood that can pass through a vessel.
Understanding the dynamic balance between pressure gradient and resistance is crucial for maintaining optimal blood flow. An ideal pressure gradient ensures that blood can reach all parts of the body with sufficient force. At the same time, minimal resistance allows blood to travel with ease. Any disruption to this delicate balance, such as increased resistance due to plaque buildup or reduced pressure gradient caused by heart failure, can compromise blood flow and have detrimental effects on overall health.