Nucleotides: The Building Blocks Of Protein Synthesis (9 Required For 3 Amino Acids)
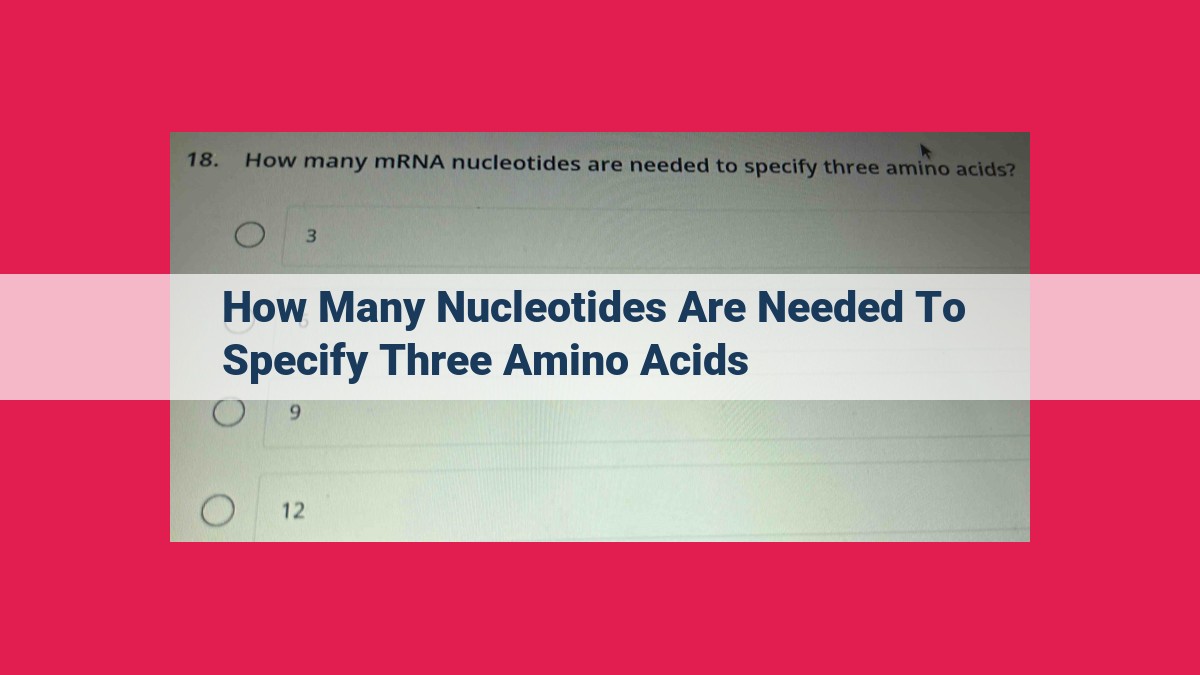
To specify three amino acids, 9 nucleotides are required. Codons, composed of three successive nucleotides, provide the genetic code for each amino acid. Since each codon comprises three nucleotides, three amino acids necessitate three codons, resulting in a total of 9 nucleotides. This relationship highlights the critical role of nucleotides in determining the amino acid sequence during protein synthesis.
Understanding the Genetic Code: A Tale of Codons and Amino Acids
The Blueprint of Life
Our DNA, the blueprint of life, holds the genetic instructions for every cell in our body. These instructions are encoded in a language known as the genetic code, which consists of codons, three-letter sequences of nucleotide bases. Each codon specifies a particular amino acid, the building blocks of proteins.
Codons: The Code’s Dictionary
Think of codons as words in a foreign language. Just as words define meaning, codons define which amino acid will be added to the growing protein chain. Each amino acid corresponds to one or more specific codons, ensuring the correct sequence of amino acids in the protein.
The Wobble Hypothesis: A Flexible Interpreter
However, the genetic code has a quirky feature known as the wobble hypothesis. The third nucleotide of a codon is sometimes less specific, allowing it to pair with multiple nucleotides in the anticodon of tRNA molecules (we’ll get to them later). This flexibility allows for more efficient protein synthesis and error prevention.
In essence, the genetic code is a complex but elegant system that translates the DNA’s instructions into the proteins that drive life’s processes. Understanding this code is essential for unraveling the mysteries of biology and advancing our knowledge of genetics and medicine.
Deciphering the Redundancy of the Genetic Code
In the intricate world of molecular biology, the genetic code orchestrates the precise assembly of life’s building blocks, amino acids. This code, encoded within the sequences of DNA, employs a clever strategy known as redundancy. Redundancy in the genetic code refers to the fascinating use of multiple codons, or three-letter sequences of nucleotides, to code for the same amino acid.
This ingenious design serves a crucial purpose. By allowing multiple codons to represent a single amino acid, the genetic code gains remarkable resilience and adaptability. This redundancy is the genomic shield of life, protecting against errors that could disrupt the intricate dance of protein synthesis.
For example, if a single mutation were to alter a codon for a particular amino acid, the genetic code’s redundancy would ensure that other codons encoding the same amino acid could still be read by the translational machinery. This flexibility provides a safety net, preventing harmful mutations from derailing protein production.
Moreover, redundancy enhances the genetic code’s capacity for evolution. As organisms adapt to changing environments, the ability to encode the same amino acid with different codons allows for subtle variations in gene expression. This subtle fine-tuning can lead to beneficial changes in protein structure and function, driving the evolutionary process.
Decoding the Genetic Blueprint: The Role of Aminoacyl-tRNA Molecules
In the realm of molecular biology, the genetic code holds the key to life’s intricate tapestry. Aminoacyl-tRNA molecules play a pivotal role in deciphering this code and transforming it into the building blocks of proteins. So, let’s embark on a captivating journey to unravel their structure, function, and the mesmerizing process of protein synthesis.
Aminoacyl-tRNA: The Bridge Between Code and Protein
Imagine an elegant dance between amino acids and their molecular messengers, known as tRNAs (transfer RNAs). Aminoacyl-tRNA molecules are like tiny bridges that transport amino acids to the protein synthesis machinery. Each tRNA has an exclusive attachment site for a specific amino acid, forming a stable complex called an aminoacyl-tRNA synthetase. These complexes are the gatekeepers, ensuring that the correct amino acids are delivered to the ribosome.
Navigating the Genetic Code
As the ribosome reads the genetic code, sequence by sequence, it relies on aminoacyl-tRNA molecules to supply the corresponding amino acids. These molecules recognize specific codons (three-nucleotide sequences) within the mRNA (messenger RNA). Each codon corresponds to a particular amino acid, and the sequence of codons determines the sequence of amino acids in the nascent protein.
Decoding the Genetic Dance
The process of protein synthesis is a breathtaking ballet of molecular interactions. The ribosome, a complex molecular machine, binds to the mRNA and begins decoding the genetic code. As each codon is read, the corresponding aminoacyl-tRNA molecule delivers its amino acid payload. The ribosome then catalyzes the formation of a peptide bond between the newly arrived amino acid and the growing polypeptide chain. Step by step, amino acid by amino acid, the protein takes shape, its structure and function dictated by the genetic code.
Ensuring Accuracy: The Wobble Hypothesis
Remarkably, the genetic code exhibits a degree of redundancy, with multiple codons often coding for the same amino acids. This redundancy is an ingenious evolutionary adaptation, reducing the chance of errors during protein synthesis. The wobble hypothesis explains this phenomenon, suggesting that the tRNA molecule can “wobble” slightly in its base pairing with the mRNA, allowing it to recognize multiple codons for the same amino acid. This molecular flexibility helps ensure that the correct amino acids are incorporated into the growing protein, even if there are slight variations in the mRNA sequence.
In conclusion, aminoacyl-tRNA molecules are the unsung heroes of protein synthesis, the essential couriers that translate the genetic code into the proteins that drive life’s essential processes. Their structure, function, and interplay with the ribosome form an intricate dance that unravels the secrets of life, one codon at a time.
The Importance of Reading Frame in Genetic Expression
In the intricate world of molecular biology, the genetic code serves as the blueprint for life, dictating the sequence of amino acids that make up proteins. This code is read in a specific manner, known as the reading frame, which is essential for the proper synthesis of functional proteins.
Defining Reading Frame
The reading frame refers to the specific set of three nucleotides that are grouped together to form a codon. Each codon corresponds to a particular amino acid or a stop signal. The ribosome, the molecular machine responsible for protein synthesis, reads the mRNA sequence in a linear fashion, starting from a specific start codon. This initial codon determines the reading frame for the entire mRNA molecule.
Consequences of Shifts in Reading Frame
Maintaining the correct reading frame is crucial for accurate protein synthesis. A shift in the reading frame, even by a single nucleotide, can have dramatic consequences. This shift causes the ribosome to group nucleotides into different codons, resulting in the incorporation of incorrect amino acids into the protein sequence.
The impact of a reading frame shift can range from subtle changes in protein structure to complete loss of function. In some cases, the shift may introduce premature stop codons, leading to the production of truncated proteins. These aberrant proteins can disrupt cellular processes, leading to genetic disorders or even cell death.
Maintaining the Correct Reading Frame
Several mechanisms exist to ensure the maintenance of the correct reading frame during translation. The start codon, typically AUG, is recognized by a specialized initiator tRNA that binds to the ribosome and initiates protein synthesis. Additionally, each codon is specifically recognized by its cognate tRNA molecule, ensuring the correct amino acid is incorporated.
Furthermore, the ribosome itself acts as a molecular ruler, ensuring that nucleotides are grouped in threes and preventing the formation of incorrect reading frames. The spacing between the tRNA molecules on the ribosome further stabilizes the reading frame, preventing shifts.
The reading frame is a fundamental aspect of genetic expression, ensuring the accurate synthesis of proteins from mRNA. Shifts in reading frame can disrupt protein structure and function, highlighting the critical importance of maintaining the correct reading frame throughout the translation process.
The Enchanting Symphony of Translation: Unraveling the Process of mRNA to Protein
In the vibrant kingdom of molecular biology, a captivating symphony unfolds as mRNA transforms into a polypeptide chain, a vital step in the creation of proteins, the workhorses of life. This process, known as translation, is a testament to the harmonious interplay between the ribosome, tRNA, and amino acids.
Let us embark on a journey to unravel this intricate dance, beginning with the ribosome, the maestro of protein synthesis. This complex molecular machine, composed of RNA and proteins, serves as a platform where the mRNA sequence is meticulously translated.
Enter the tRNA, the versatile messengers of amino acids. Each tRNA molecule carries a specific amino acid, which it delivers to the ribosome in accordance with the genetic code. Like tiny gondolas on the canals of Venice, tRNAs navigate the mRNA sequence, their anticodon pairing with the complementary codon of the mRNA.
As the ribosome scans the mRNA sequence, it undergoes a mesmerizing ballet. Step by step, it recognizes each codon and invites the corresponding tRNA molecule to the dance. The amino acid carried by the tRNA is then linked to the growing polypeptide chain, forming a bond that will ultimately determine the protein’s shape and function.
This process of codon recognition and amino acid linkage repeats itself until the ribosome reaches a stop codon, signaling the end of the translation. The newly synthesized polypeptide chain, still a jumble of amino acids, undergoes further modifications to achieve its mature and functional form.
Through the meticulous choreography of translation, the genetic code embedded in mRNA becomes manifest, giving rise to the diverse array of proteins that sustain life. It is a symphony of molecular precision and artistry, laying the foundation for countless biological processes and shaping the very fabric of our existence.
Calculating Nucleotides for Three Amino Acids: Unraveling the Genetic Code
In the intricate tapestry of life, the genetic code plays a pivotal role, dictating the sequence of amino acids that give rise to the proteins essential for our existence. Nucleotides, the building blocks of DNA and RNA, form the foundation of this code. Understanding the relationship between nucleotides and amino acids is crucial for deciphering the genetic blueprint.
Imagine a scenario where we want to specify the order of three amino acids: alanine, valine, and serine. The genetic code utilizes codons, sequences of three nucleotides, to instruct the synthesis of specific amino acids. Each codon corresponds to a particular amino acid, providing an intricate language for translating genetic information into the building blocks of proteins.
To calculate the number of nucleotides required for these three amino acids, we simply multiply the number of codons by three. Each codon consists of three nucleotides, so for three amino acids, we need a total of 9 nucleotides. This simple formula highlights the remarkable efficiency of the genetic code.
Consider the following example:
- Alanine: GCA
- Valine: GUU
- Serine: UCU
To specify these three amino acids, we concatenate their respective codons: GCA + GUU + UCU = GCAGUUCU. This sequence of 9 nucleotides carries the genetic instructions for assembling the desired amino acid sequence.
Understanding the relationship between nucleotides and amino acids empowers us to decipher the genetic code and unravel the mysteries of life.