Neon’s Electronic Structure: Understanding Electron Configuration And Chemical Properties
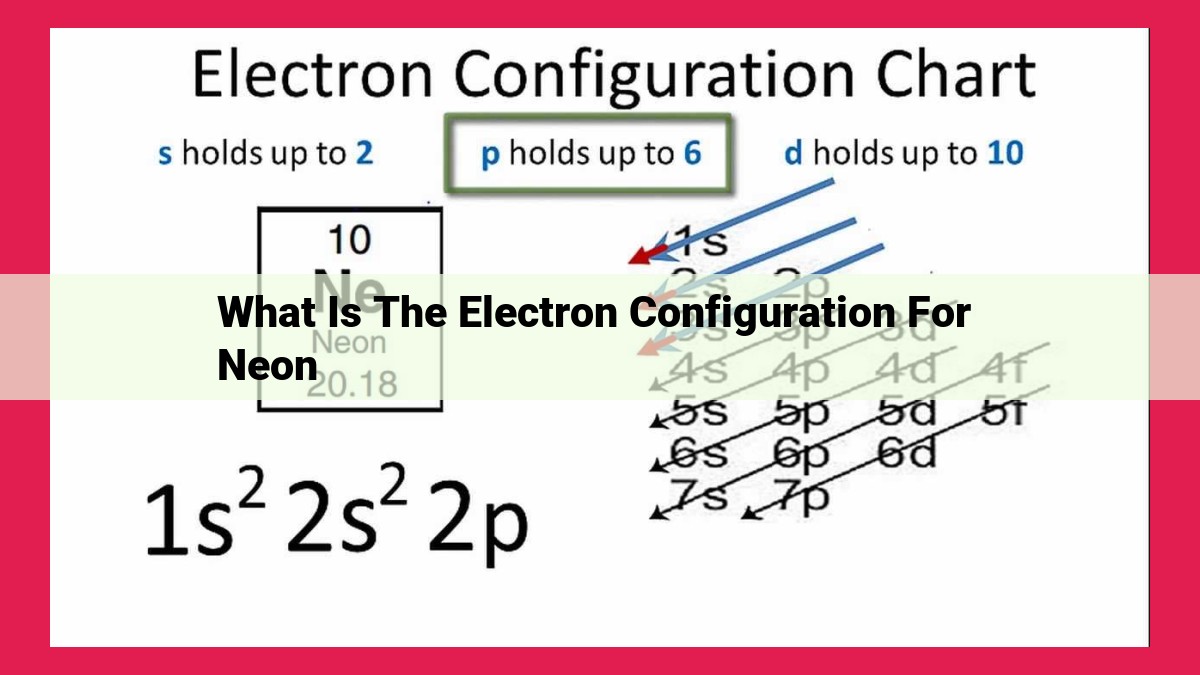
Neon’s electron configuration, 1s² 2s² 2p⁶, is determined by its atomic number (10) and follows specific principles: the Aufbau principle guides electron filling in orbitals, the Pauli exclusion principle limits electrons per orbital, and Hund’s rule favors maximum spin multiplicity. Neon’s noble gas configuration, with a full outermost electron shell, contributes to its stability and inert chemical properties.
Electron Configuration: Unraveling the Secrets of Atomic Structure
Every atom is a miniature universe, teeming with subatomic particles. Among these, electrons play a pivotal role in shaping the identity and behavior of an atom. Their arrangement within an atom, known as its electron configuration, holds the key to understanding its chemical properties and reactivity.
Electron configuration is the distribution of electrons across various energy levels and sublevels within an atom. It reveals the number and arrangement of electrons in each sublevel, providing insights into the atom’s overall structure and stability. Understanding electron configuration is essential for predicting the chemical reactions and bonding behaviors of elements, unlocking the mysteries of the atomic realm.
Atomic Number: The Gateway to Electron Configuration
In the realm of atomic structure, there exists a fundamental concept known as atomic number. It serves as a defining characteristic of an element, dictating the arrangement of electrons within its electron cloud. Understanding atomic number is crucial for comprehending the chemical behavior and properties of elements.
Every atom possesses a unique atomic number, which is represented by the symbol Z. It is a number that signifies the total number of protons present in the atom’s nucleus. Protons carry a positive electric charge, contributing to the positive charge of the nucleus.
The atomic number is directly related to the number of electrons an atom contains. In a neutral atom, the number of electrons is equal to the atomic number. This balance ensures that the positive charge of the protons is counterbalanced by the negative charge of the electrons, resulting in an overall neutral charge for the atom.
The atomic number serves as a crucial factor in determining the electron configuration of an atom. Electron configuration refers to the distribution of electrons across various energy levels and orbitals within the atom. The atomic number dictates the number of electrons, which in turn influences the arrangement and behavior of these electrons.
By understanding atomic number and its relationship with electron configuration, we gain a deeper insight into the fundamental building blocks of matter. This knowledge forms the foundation for exploring the chemical properties and reactivity of elements, enabling us to comprehend the diverse phenomena that shape the world around us.
Electron Configuration Notation: Unraveling the Secrets of Atomic Structure
In the fascinating realm of atomic structure, electron configuration plays a pivotal role in deciphering the arrangement of electrons within an atom. Picture an atom as a miniature solar system, with electrons orbiting the nucleus like planets. Electron configuration provides us with a detailed roadmap of these electron orbits.
To grasp this concept, let’s delve into the standard notation used to represent electron configurations. Each electron is assigned a unique address, consisting of three parts:
-
Energy levels (n): These are concentric shells surrounding the nucleus, labeled as 1, 2, 3, and so on, with increasing distance from the nucleus.
-
Sublevels (l): Within each energy level, electrons occupy distinct sublevels designated as s, p, d, and f. Each energy level can accommodate a limited number of sublevels.
-
Orbitals (ml): Sublevels are further divided into orbitals, which are the specific spatial regions where electrons are most likely to be found. Each orbital can hold a maximum of two electrons.
To write the electron configuration, we use a combination of these three identifiers. For example, the electron configuration of the first energy level is written as 1s², indicating that it has two electrons in the s orbital.
Understanding electron configuration is crucial because it sheds light on the chemical properties of elements. Elements with similar electron configurations often share similar chemical behaviors, as the outer electrons are the ones that participate in chemical reactions. Electron configuration also enables us to predict the stability and reactivity of atoms.
The Aufbau Principle: Guiding Electron Distribution in Atoms
In the intriguing realm of atomic structure, understanding electron configuration is essential for comprehending the behavior and properties of elements. The Aufbau Principle provides a crucial guide in this endeavor, shedding light on how electrons occupy orbitals within an atom.
The Aufbau Principle, formulated by Niels Bohr and Wolfgang Pauli, states that electrons fill atomic orbitals in order of increasing energy. This means that electrons prefer to occupy lower energy orbitals before filling higher energy ones. Orbitals are conceptualized as regions surrounding the nucleus where electrons are likely to be found. Each orbital is characterized by its energy level and is designated by a unique set of quantum numbers.
The energy levels of orbitals are determined by their distance from the nucleus and their shape. Orbitals closer to the nucleus have lower energy, while those farther away have higher energy. Additionally, the shape of an orbital affects its energy level, with orbitals of different shapes having different energy levels. For example, s orbitals, which are spherical in shape, have lower energy than p orbitals, which have a dumbbell shape.
The Aufbau Principle suggests that electrons first fill s orbitals, followed by p orbitals, then d orbitals, and so on. Within each energy level, electrons fill orbitals with different spins, a property known as electron spin. Electrons with opposite spins can occupy the same orbital, but no two electrons in the same atom can have the identical set of four quantum numbers, as dictated by the Pauli Exclusion Principle.
The Aufbau Principle is crucial for determining the electron configuration of atoms. By knowing the atomic number, the number of protons in the nucleus, and employing the Aufbau Principle, scientists can predict the arrangement of electrons in an atom’s orbitals. This knowledge provides essential insights into an element’s chemical behavior and its position on the Periodic Table.
Unveiling the Enigma of Electron Configuration: The Pauli Exclusion Principle
In the realm of atomic structure, electrons play a pivotal role, and their arrangement, known as electron configuration, holds immense significance. One of the fundamental principles governing electron configuration is the Pauli Exclusion Principle.
Imagine a lively party where no two guests can occupy the same seat – that’s essentially the essence of the Pauli Exclusion Principle. It postulates that in an atom, no two electrons can have the same set of four quantum numbers.
What’s a Quantum Number?
Quantum numbers are the address codes for electrons within an atom. They specify the energy level, shape, orientation, and spin of electrons.
Implications for Electron Configuration
The Pauli Exclusion Principle has a profound impact on electron configuration. It dictates that electrons within an atom must differ in at least one of their quantum numbers. In other words, they cannot be identical twins.
Electron Spin
One crucial factor differentiating electrons is their spin. Electrons can either spin clockwise or counterclockwise, like tiny spinning tops. The Pauli Exclusion Principle demands that electrons in the same orbital must have opposite spins.
Applications of the Pauli Exclusion Principle
This principle plays a pivotal role in explaining various atomic phenomena. It determines the maximum number of electrons that can occupy a particular energy level and explains the aufbau principle, which guides electron filling in orbitals.
Implications for Neon’s Electron Configuration
For instance, consider the element neon, with an atomic number of 10. Based on the Pauli Exclusion Principle, its electrons must be arranged in the following way:
1s² 2s² 2p⁶
This configuration reflects the fact that the first energy level can hold a maximum of two electrons with opposite spins, and the second energy level can hold a maximum of eight electrons with different spin orientations.
Why it Matters
The Pauli Exclusion Principle is not just a technicality; it has real-world implications. It determines the stability of atoms and influences their chemical properties, explaining why certain elements form specific types of bonds and behave in predictable ways.
So, next time you hear about electron configuration, remember the Pauli Exclusion Principle – the rule that ensures no two electrons in an atom are identical, like guests at a party claiming the same seat.
Hund’s Rule: Maximizing Electron Unpaired Status
In the fascinating realm of atomic structure, Hund’s Rule emerges as a crucial principle that dictates electron behavior and influences the electron configuration of atoms. Let’s delve into this fascinating rule and uncover its implications for the organization of electrons within atoms.
Unveiling Hund’s Rule
Hund’s Rule, formulated by the renowned physicist Friedrich Hund, asserts that when multiple electrons occupy orbitals of equal energy (degenerate orbitals), they tend to occupy separate orbitals with parallel spins. This phenomenon is driven by the minimization of electron-electron repulsion.
In other words, electrons strive to maintain a maximum distance from one another by distributing themselves across degenerate orbitals with their spins aligned in the same direction. This arrangement maximizes the atom’s overall spin multiplicity, which is the number of unpaired electrons.
The Concept of Spin Multiplicity
Electron spin, a fundamental property of electrons, refers to their intrinsic angular momentum. Electrons can either spin clockwise or counterclockwise, giving rise to two possible spin states, +1/2 and -1/2.
Spin multiplicity, denoted by the symbol 2S+1, quantifies the number of unpaired electrons in an atom. A higher spin multiplicity corresponds to a greater number of unpaired electrons and, consequently, a higher energy state.
Implications for Electron Configuration
Hund’s Rule plays a significant role in determining the electron configuration of atoms. It dictates the filling of orbitals such that electrons occupy degenerate orbitals with parallel spins before pairing occurs.
This rule has profound implications for the chemical properties of elements. Atoms with high spin multiplicities, characterized by a larger number of unpaired electrons, often exhibit paramagnetic behavior, meaning they are attracted to magnetic fields.
Example: The Intriguing Case of Neon
Let’s consider the electron configuration of neon, an element with an atomic number of 10. According to Hund’s Rule, the electrons in neon’s 2p subshell, which has three degenerate p orbitals, will occupy separate orbitals with parallel spins. This configuration, 1s² 2s² 2p⁶, gives neon a closed-shell configuration with a spin multiplicity of 1 (2S+1 = 3).
Hund’s Rule, a cornerstone of atomic physics, provides a crucial framework for understanding electron configuration and the organization of electrons within atoms. By maximizing spin multiplicity and minimizing electron-electron repulsion, Hund’s Rule plays a pivotal role in determining the chemical properties of elements and shaping the world of chemistry as we know it.
Noble Gas Configuration: A Tale of Stability and Chemical Inertia
In the vast atomic realm, certain elements possess a unique electron configuration that grants them exceptional stability: noble gas configuration. This special arrangement, with a full outermost electron shell, has a profound impact on an atom’s chemical behavior.
Just as humans seek contentment in life, atoms strive for a stable electron configuration. This stability is achieved when the outermost electron shell, known as the valence shell, is filled to its capacity. Noble gases, like helium, neon, and argon, are the epitome of this stability, possessing a full valence shell and displaying a remarkable reluctance to engage in chemical reactions.
This inertness can be attributed to the tight grip these elements have on their valence electrons. With a full complement of electrons, they lack the driving force to form chemical bonds with other atoms. In contrast, elements with incomplete valence shells, such as sodium or chlorine, readily participate in chemical reactions to satisfy their electron cravings.
The stability of noble gas configuration is a fundamental pillar of our understanding of atomic structure and chemical bonding. It explains why noble gases exist in their elemental form, undisturbed by the allure of chemical interactions. This exceptional stability also provides the foundation for the concept of the octet rule, which governs the electron configurations of many stable molecules and ions.
In the following section, we will delve into the electron configuration of neon, a quintessential example of a noble gas. We will apply the principles of atomic structure discussed earlier to unravel its electron arrangement and explore its implications for neon’s remarkable stability and inertness.
Electron Configuration of Neon: Unlocking the Secrets of Atomic Structure
In the vast tapestry of the atomic world, electron configuration plays a pivotal role in deciphering the intricate details of matter. It unveils the arrangement of electrons within an atom, offering insights into its stability, reactivity, and chemical properties. To unravel these secrets, let’s embark on a journey to unravel the electron configuration of neon, a noble gas known for its remarkable stability.
Neon: A Peek into Its Atomic Makeup
Neon occupies the 10th position in the Periodic Table, boasting an atomic number of 10. This number signifies the total count of protons residing in the atomic nucleus, which in turn determines the number of electrons orbiting around it. In the case of neon, the number of electrons matches the number of protons, leading to a neutral electrical charge.
The Building Blocks of Electron Configuration
To grasp electron configuration, we must delve into the fundamental concepts of orbitals, sublevels, and quantum numbers. Orbitals represent the three-dimensional spaces where electrons can be located. Each orbital is characterized by a unique set of quantum numbers, including the principal quantum number (n), azimuthal quantum number (l), and magnetic quantum number (ml). These quantum numbers dictate the energy level, shape, and orientation of the orbital.
Electron Configuration Notation: A Guide to Order
Electron configuration is represented using a standardized notation that captures the distribution of electrons among various orbitals. The notation follows a simple format:
1s² 2s² 2p⁶
In this example, the numbers (1, 2) represent the principal quantum number, n, indicating the energy level of the orbitals. The letters (s, p) denote the azimuthal quantum number, l, which describes the orbital’s shape. The superscripts (² and ⁶) indicate the number of electrons occupying each orbital.
Unveiling Neon’s Electron Configuration
Applying the principles of electron configuration, we can construct the electron configuration for neon, which is:
1s² 2s² 2p⁶
This notation reveals that neon’s electrons are distributed as follows:
- Two electrons reside in the 1s orbital (n = 1, l = 0)
- Two electrons occupy the 2s orbital (n = 2, l = 0)
- Six electrons are present in the 2p orbital (n = 2, l = 1)
Stable and Inert: The Significance of Neon’s Configuration
Neon’s electron configuration holds immense significance, contributing to its stability and inertness. The outermost electron shell (n = 2) is completely filled with eight electrons, a configuration known as the noble gas configuration. This arrangement confers exceptional stability, making neon reluctant to participate in chemical reactions. The full outermost shell effectively shields the inner electrons, minimizing their interaction with other atoms.
The exploration of neon’s electron configuration has unveiled the intricacies of atomic structure. Through this understanding, we appreciate the fundamental principles governing electron distribution and the stability of noble gases. Electron configuration serves as a crucial tool for unraveling the chemical behavior of elements, shaping the world around us in myriad ways.